In the first part of this survey (“Foodborne Parasites: An Insidious Threat to Food Safety and Public Health”), we looked in depth at common pathogenic parasites behind foodborne illness outbreaks and assessed the extensive geography of their origin and prevalence. In this concluding part, we look in detail at industry and regulators’ approaches to preventive control and eradication in response to this expansive threat to the global food supply system and its consumers.
Many researchers, food safety scientists, and others have referred to foodborne parasites as an invisible threat. Our research suggests that the only reason they are invisible is our refusal to see them. It is an irrefutable truth that medically important foodborne parasites are a serious threat to public health. It has been estimated that humans harbor some 300 species of parasitic worms and some 70 or more species of protozoa.1 Not all parasites are foodborne. It is also good fortune for public health that many of the medically important parasites—those that infect and cause disease in humans or animals—are rare in the natural world. Since food safety science commonly relegates parasitology to the status of a subspecialty of microbiology, the medically important parasites are sometimes considered only in the context of tropical medicine, despite abundant evidence confirming their prevalence in the food supply, far beyond the tropics.
Ireland’s public health officials have recently reported a huge spike in Cryptosporidium infections there. The country’s Health Service Executive-Health Protection Surveillance Centre (HPSC) data show there had been 428 reports of cryptosporidiosis up to mid-April 2021, compared with 148 in the same period in 2020.2 The majority of the illnesses were reported in people under the age of 14. The HPSC have not confirmed the mode of infection or directly linked its occurrence to food, but the agency has not ruled out the possibility that food or water is the culprit. The HPSC notes that this parasite can be transmitted by contact with infected farm animals, contaminated water, food, and dairy products, or as a result of poor hand hygiene.
In an April 2021 published paper from Dartmouth College, public health archaeologists report the discovery of helminth eggs, whipworms, and tapeworms in 200-year-old human feces recovered from a long-defunct New England outhouse.3 According to the paper, the privy was formerly owned and used by a wealthy, well-educated, rural family. This important finding shows how widespread parasitic infections were in early American society. It had been assumed for this era, according to the Dartmouth researchers, that intestinal parasites were a public health issue ostensibly for impoverished urban dwellers due to poor sanitation, poor hand hygiene, and overcrowding in the densely populated cities.
In an even more recent (June 23, 2021) report from the Norwegian Scientific Committee for Food and Environment, it was confirmed that Toxoplasma gondii tops a risk-ranking report of 20 pathogens in Norway.4 That report also shows the parasitic flatworm Echinococcus multilocularis ranked third in this risk assessment. Hence, two of Norway’s top five high-risk pathogens are foodborne parasites.
Medically important parasites are associated with a broad array of foods, as well as a majority of the global food supply chains. Foodborne parasites are transmitted by meat and meat foods, as well as by fresh fruits, vegetables, and seafood. The risk of exposure to these parasites varies greatly by geographic region. Africa, Asia, and other tropical regions are hot spots, but most other regions have specific challenges from certain worms and protozoa that can cause illness and disease in humans.5 Foodborne parasites contribute greatly to the global public health burden.
Recent data from the U.S. Centers for Disease Control and Prevention (CDC) confirm a public health burden due to foodborne disease of some 48 million cases in the U.S., with 128,000 hospitalizations and upward of 3,000 deaths annually.6 Data from the World Health Organization (WHO) on the global impact of foodborne disease (for 2010) report 600 million illnesses and 420,000 deaths. The WHO report states further that the preponderance of this public health burden was due to diarrheal disease agents, particularly norovirus and Campylobacter spp.7 Foodborne parasites also contribute tremendously and insidiously to the public health and disease burden. A 2015 WHO report confirms that the parasite Taenia solium is among the major causes of foodborne disease deaths globally. WHO estimates that some 1.5 billion people were infected with intestinal worms in 2018, predominantly in disadvantaged communities.8
Different types of parasitic diseases can be transmitted to humans by an assortment of food materials. Pork, freshwater crustaceans, sheep, fish, fresh fruit, and vegetables have been implicated in disease outbreaks. Water and the natural environment also have been shown to be high-risk routes of infection for both humans and farm animals. Foodborne parasites affect the health of millions of people every year, infecting muscle tissue and organs, causing epilepsy, anaphylactic shock, amoebic dysentery, and other problems.9 Many parasites can live in the human body for decades. Despite the huge social costs and global impacts, information is generally lacking regarding just where these parasites come from, how they live in the human body, and—most importantly—how they make us sick.
In 2014, WHO/FAO published a multicriteria-based ranking of risk for the management of foodborne parasites. As noted in the first part of this survey, the WHO/FAO profile for the top 10 foodborne parasites and food sources includes:10
- Taenia solium (pork tapeworm): Pork
- Echinococcus granulosus (hydatid worm or dog tapeworm): Fresh produce
- Echinococcus multilocularis (a type of tapeworm): Fresh produce
- Toxoplasma gondii (protozoa): Meat from small ruminants, pork, beef, game meat (red meat and organs)
- Cryptosporidium spp. (protozoa): Fresh produce, fruit juice, milk
- Entamoeba histolytica (protozoa): Fresh produce
- Trichinella spiralis (pork worm): Pork
- Opisthorchiidae (family of flatworms): Freshwater fish
- Ascaris spp. (small intestinal roundworms): Fresh produce
- Trypanosoma cruzi (protozoa): Fruit juices
Controlling the transmission of these parasites is a great challenge for the food processing industry, as well as for those charged with regulating the industry and protecting public health. With the advent of Hazard Analysis and Critical Control Points (HACCP) and its adoption globally as the preferred food safety management strategy, the food industry is now compelled to conduct hazard analysis (risk assessment) for their products and manufacturing processes. These qualitative or quantitative assessments of risk are fundamental to food safety and are essential for developing the programs, practices, and procedures that are indispensable for mitigating foodborne threats. The Critical Control Point (CCP) of a food safety plan is its aegis to keep the identified hazard from becoming an actual contamination event. The critical limits, which subordinate and define a CCP, are the minimum performance criteria necessary to control the identified hazard. The critical limits also reflect the degree of risk tolerance for the specific hazard, in terms of expected public health outcomes. Let us be clear and unequivocal on this point: There is no such thing as zero risk in the food industry, and anyone chasing zero risk is running the proverbial fool’s errand.
The concept of the food safety objective (FSO) has emerged internationally as a means for expressing an acceptable level of health protection (ALOHP) for consumers. For example, there continues to be much debate as to what constitutes an acceptable level of protection (risk) for Listeria monocytogenes in ready-to-eat meat products. Many years ago, Canada established an action level of 100 CFU/g for this category of food.11 Their American neighbors, however, disagree and continue with a policy of zero tolerance for L. monocytogenes in ready-to-eat products.12 Similarly, the accepted roundworm burden for certain species of fish, according to the UK’s model purchase specification, is established as a maximum of three cod worms in 3.2 kg (7.1 pounds) of fillets of whitefish, as judged by visual examination.13 An international standard for roundworms in fish, Codex Alimentarius, allows a maximum of five worms in 1 kg (2.2 pounds) of fish of certain species.14 Discordant though they may be, these standards and specifications are excellent exemplars of the ALOHP and FSO concepts.
Pathogenic Parasites Pose a Risk Along Entire Supply Chain
Epidemiologic studies have shown that parasite contamination of food and food ingredients may occur at several points along the supply chain, starting from the use of contaminated water for irrigation and contact with animal or human excrement during cultivation to contaminated harvesting equipment. It may also include unhygienic practices of the farmworkers in the field or others handling the food material prior to consumption. The challenge, for food safety assurance, is to provide validated mitigation methods targeting control of these exceedingly complex pathogens.
Across the vast expanse of the global food processing industry, conventional pathogen control methods include such techniques as washing, blanching, pasteurization, sterilization, pH and water activity control, freezing, irradiation, fumigation, culling, and inspection. Obviously, all categories of human food are not compatible with all types of preventive control options. Preventive control is, in fact, product and process specific. Low-acid canned foods require a sterilization process (F0 = 3 min), whereas acidified canned foods, pH <4.6, may be rendered safe by pasteurization (F16200 = 10 min), for example. Control of foodborne parasites in seafood is by necessity different from the control measures applied to fresh fruit and vegetables or animal flesh. What follows is an overview of foodborne parasite control measures and programs currently used by industry and regulatory agencies. It includes discussion of novel methods that may also prove effective for mitigating foodborne parasites.
Meat and Poultry Products
Interrupting the life cycle of parasites to prevent them from becoming established in food animals or other food materials is a time-tested control strategy with excellent public health outcomes. The incidence of Trichinella spp., for example, in the U.S. swine population has been dramatically reduced due to on-farm interventions that disrupt the organism’s life cycle and prevent its transmission to either an intermediate or terminal host. Since the passage of the Federal Meat Inspection Act in 1906, the U.S. Department of Agriculture (USDA) has been an active participant with industry in ensuring the safety of meat and meat-containing food products. Mandatory inspections by the USDA’s Food Safety and Inspection Service (USDA-FSIS) for meat and poultry offered in interstate commerce are the fulcrum of the food safety control efforts for America’s meat and poultry industries. Federal meat inspectors are formally trained veterinarians. They are charged with identifying and removing defective and or infected animals from processing operations, before and after slaughter. These inspections include examination of the animals for the presence of medically important parasites. The inspectors are also vested with vast enforcement powers, including suspending operations at a processing facility when Sanitation Standard Operating Procedures violations or other objectionable conditions or practices are observed.
Between 1947 and 1967, CDC surveillance data show a precipitous overall decline in swine-mediated trichinosis in the U.S.15 Public health officials attribute the decline of this pernicious, parasitic disease in humans to changes in on-farm practices related to housing and feeding and to implementation of improved standards of care for swine prior to slaughter. Farmers were advised against feeding the animals garbage and counseled to shield the pigs from exposure to rats—an important vector in the transmission of the disease. Vaccines and other veterinary interventions have also proven effective in controlling the spread of the parasite in hog farming operations. USDA-driven public education on the perils of eating undercooked pork has also helped mitigate the occurrence of swine-mediated trichinosis.
By contrast, meat and poultry products that are not intended for interstate commerce are not so rigorously regulated. In fact, they are exempt from most federal regulation. In this circumstance, state public health authorities hold purview and are therefore responsible for meat inspection activities occurring within the state’s boundaries. This introduces a vulnerability in the defense against foodborne parasitic infection. In the absence of federal oversight, there is potential for great variability among states in how the inspections are conducted.
A further complication, exacerbating an already complex public health morass, are the unregulated sources of meat. Animals raised and slaughtered for personal consumption, as well as the meat from game animals such as feral pigs, bears, or deer, are largely unregulated. Therefore, this category of unregulated meat sources represents an extraordinary public health threat for the potential transmission of foodborne parasites. Bears and feral pigs are well-known reservoirs for Trichinella. The threat finds its way to consumers in a peculiar way: a taste for lightly cooked meat. Compare this situation with poultry, which has a similar risk of parasitic infection. Poultry now accounts for greater than 30 percent of all meat consumed worldwide. According to Ruff (1999), “…parasites are a problem wherever poultry are raised, whether in large commercial operations or in small back-yard flocks.”16 The good news, from a public health perspective, is that poultry is seldom if ever eaten raw or undercooked; whereas anecdotal evidence seems to suggest a trend toward eating pork that has been lightly cooked—that is, served medium rare.
The comprehensive, integrative approach to food safety, involving all high-risk nodes along the forward (toward the consumer) supply chains, as used by the USDA-FSIS for controlling pathogens, bacterial and parasitic, in meat and poultry products, is an excellent management strategy that has yielded positive public health outcomes for many years (see Figure 1). Under the USDA’s Swine Control Program, countermeasures are deployed on the farm, in the slaughtering operations, at retail, and also at the point of use by the consumer.
Ultimately, the USDA has published cooking guidelines that can be seen as the definitive point-of-use preventive control measure. The agency advises cooking pork to an internal temperature, at the coolest part of the oven, greater than 60 °C (140 °F) and maintaining that temperature for a minimum of 5 minutes to inactivate parasites. It should be noted, however, that because there may be other more heat-resistant pathogenic organisms occurring in pork, the WHO/FAO recommends a minimum internal cooking temperature of 71 °C (160 °F).5 Another effective and widely adopted preventive control measure for parasitic pathogens is freezing pork at –10 °C (14 °F) or lower and holding it frozen for a minimum of 10 days.5 This control has been validated and confirms, with a high degree of confidence, that the parasites within the meat are completely inactivated.
U.S. Food and Drug Administration (FDA) regulations also permit the use of ionizing radiation [21 Code of Federal Regulations §179.26 (b)(1)] at a maximum absorbed dose of 1 kGy for the control of Trichinella spiralis in pork carcasses or fresh, non-heat-processed cuts of pork carcasses.
Consumers have a very important role for ensuring the safety of such products, which is codified in federal regulations (9 C.F.R. §317.2). This is especially true for the control of foodborne pathogens, both bacterial and parasitic. The USDA’s safe-handling instructions offer safe storage, preparation, cooking, and holding guidance that is intended to promote and ensure food safety. Like industry, the agency recognizes the consumer as a critical control point in the meat supply chain.
FIGURE 1. Modes for Controlling Foodborne Parasites
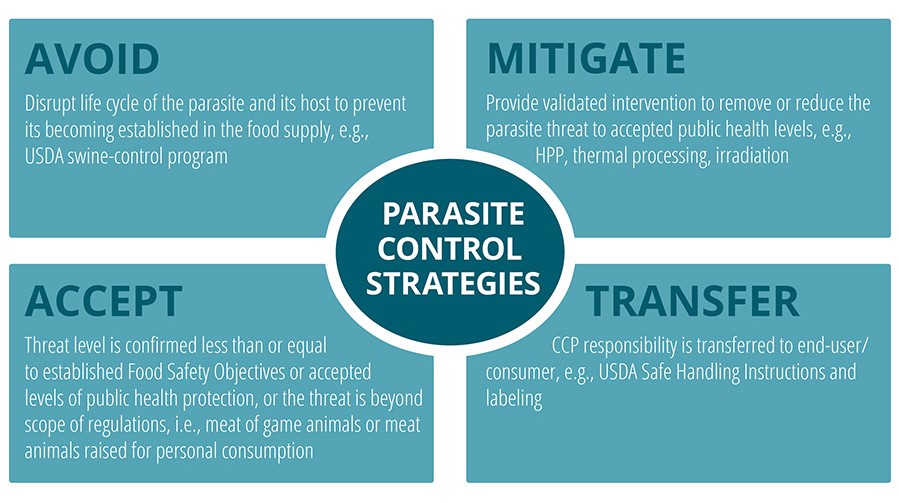
Seafood and Shellfish
Seafood HACCP regulation (21 C.F.R. §123) was first mandated by FDA in 1997 to address biological hazards in seafood, which include toxic algae in addition to pathogenic parasites.
According to FDA’s Fish and Fishery Products Hazards and Controls guidance document (chapter 5), numerous parasites should be considered in developing a food safety plan for seafood and seafood products. FDA’s high-risk list includes medically important helminths of the following classes: nematodes, cestodes, and trematodes. The agency lists the following worms as the parasites of most concern for seafood: Anisakis spp., Pseudoterranova spp., Eustrongyldies spp., Gnathbostoma spp., Diphyllobotbrium spp., Chlornorchis sinensis; Paragonimus spp., Heterophytes spp., Nanophytes salmincola, and Metagonimus spp.
Seafood products that have been implicated in human parasitic infections include: ceviche, lomi-lomi, poisson cru, herring roe, sashimi, sushi, drunken crabs, cold smoked fish and undercooked grilled fish. FDA also recommends preventive controls during the processing of any parasite-containing species of fish intended for raw consumption.
Owing to the delicate and heat-labile nature of fish and seafood products, preventive control options are often limited. Freezing, heating, inspection, and culling are the primary modes of control. Of these, only heating and freezing can be validated as suitable for use as critical control points in a food safety plan.
Freezing is by far the most efficacious and also the most widely used of the preventive control options available to the seafood industry. The effectiveness of freezing for inactivating parasites in seafood and fish products depends on several factors including the temperature of the freezing process, the length of time required to completely freeze the fish flesh, the elapsed time at which the fish is held in the frozen state, and of course the physiological status of the target parasite. This process-critical information is vital in deciding what parameters are used for the freezing and holding processes. FDA advises freezing at –4 °F (–20 °C) for seven days or freezing at –31 °F (–35 °C) or below and holding for 15 hours or freezing at –31 °F until solid and then holing the solid frozen fish at a temperature below –4 °F for a minimum of 24 hours, as sufficient for the inactivation of freeze-resistant parasites. FDA reports, for example, that cestodes (tapeworms) are more susceptible to freezing than are nematodes (roundworms), and trematodes (flukes) appear more resistant to freezing than do roundworms. Thus, a freezing process designed to eliminate flukes would also be expected to eliminate both cestodes and nematodes. That said, these processes are product and process-specific. The process parameters used for an exceptionally large fish, for example, might need to be adjusted to reflect its exceptional size.
Trimming, candling, pickling, and brining are additional control techniques frequently used by the seafood industry. These methods are not well suited as preventive controls because they cannot be validated to reliably reduce the parasite load to safe and accepted public health levels. Nematode larvae, for example, have been shown capable of survival for up to 28 days in an 80° salinometer brine (21 percent salt by weight).5 Candling, the process of manually removing the parasite, has similar limitations in that it does not effectively eliminate the hazard or minimize the parasite threat to acceptable levels. The same is true for trimming the belly flap of infected fish, a common practice in the industry. The FAO/WHO report that almost all Anisakis in fish flesh are found in the belly flaps; it follows that trimming off and discarding flaps will remove most of the worms. The greater the area of flap discarded, the greater will be the proportion of worms removed.17 However, belly-flap trimming is not seen as a reliable or effective preventive control method consistent with HACCP requirements.
Heating processes such as canning, hot smoking, or cooking, when properly applied, are excellent control measures. Cooking to achieve core temperatures between 60 °C (140 °F) and 75 °C (167 °F), with a holding time at those temperatures of between 15 and 30 minutes, is reported to inactivate parasites in most food matrices including fish and seafood.5
Fresh Fruit and Fresh Vegetables
In 2006, a multistate outbreak of E.coli O157:H7 infections were traced to fresh-cut spinach. In 2018, an even larger outbreak occurring in 29 states, involving the same organism, was reported for romaine lettuce. Another massive O157:H7 outbreak reported for leafy greens in the U.S. occurred during the autumn of 2020. In their investigation of the 2018 and 2020 outbreaks, CDC and FDA investigators have opined that the mechanism of contamination involved rainwater runoff from cattle feedlots onto farmland or into the watershed that was used for crop irrigation. FDA and CDC suggest that the water was contaminated with cattle feces that harbored the bacterial pathogen. The proposed method of contamination in these cases bears on this discussion of foodborne parasites. If this mode of contamination is viable for bacterial pathogens, it is also a legitimate means for introducing medically important parasites into the food supply. Animal feces are a potentially rich source for many of the important foodborne parasites.
Studies have shown that Ascaris lumbricoides, Cryptosporidium spp., Entamoeba histolytica, Enterobius vermicularis, Fasciola spp., Giardia intestinalis, hookworm, Hymenolepis spp., Taenia spp., Trichuris trichiura, and Toxocara spp. can infect humans who consume contaminated, uncooked, or improperly washed vegetables and fruits.18 Cyclospora spp. has also been associated with several important foodborne disease outbreaks in berries, cilantro, and other items of fresh produce. Numerous public health studies have been conducted to evaluate the role of raw vegetables in the transmission of intestinal parasites, and all have shown the importance of fruits and vegetables, particularly when consumed raw and unwashed, in the transmission of medically important parasites. Infections with important foodborne parasites (intestinal helminths and protozoa) are closely linked with conditions of poverty, unsafe water, poor agricultural practices, and lack of sanitation and hygiene.19 Many of the listed parasites are stable in the environment for long periods and capable, therefore, of survival on contaminated produce across international supply chains. The Cyclospora outbreaks in the U.S. in 2012, 2013, and 2018 were associated with fruit and vegetables that were reported to have originated in Central America, for example.20
Conventional Disinfection and Sanitation Measures
Erecting effective preventive control measures for foodborne pathogenic parasites in this category of foods is especially challenging. Conventional methods such as thermal treatments exceeding 60 °C (140 °F), for example, are not well suited for fresh produce or fresh fruits. Conventional pasteurization (72 °C [162 °F], 15 s) inactivates parasites in most foods and food ingredients. This treatment would result in great damage to most fresh-cut produce. Likewise, freezing is also not a good option for fresh produce. Freezing can be a valid option for individually quick-frozen fresh fruits and vegetables depending on the target parasite. It is important, however, to remember that certain important parasitic pathogens are resistant to freezing.
Codex’s Code of Hygienic Practices for Fresh Fruits and Vegetables (CAC/RCP 53-2003) notes that washing also has limitations because the oocysts of many medically important foodborne parasites are sticky and difficult to remove by washing alone. Moreover, the surface characteristics of some fruits and vegetables protect the parasites during washing. Consider the morphology of a head of lettuce, and then perhaps it is possible to imagine the challenge associated with penetrating and cleaning such a complex structure. In an attempt to overcome this difficulty, some researchers have studied the use of high-power ultrasound to enhance the penetrating ability of the wash water and sanitizer deeper into the interstices of the produce. The results have been mixed.21
Parasites generally survive chemical disinfection, thus making chemical applications, at effective concentrations, often unsuitable at an industrial scale. The efficacy of the washing process depends on the parasite species, its developmental stage, the specific conditions of the target food, and application conditions. Aqueous ozone and chlorine dioxide are the most promising in terms of efficacy and dosage; nevertheless, challenges remain in their application, especially for the most fragile produce. Chlorine continues to be the most widely used sanitizer in the category. FDA and the U.S. Environmental Protection Agency have also approved peracetic acid, an equilibrium mixture of hydrogen peroxide and acetic acid, to sanitize food contact surfaces of equipment and for direct washing of fruits and vegetables.
Commercial peroxygen-based disinfectants containing hydrogen peroxide, plus either peracetic acid or silver nitrate, have been tested and shown capable of inactivating Cryptosporidium parvum oocysts. The researchers report that these compounds may be useful for on-farm treatments for soil and farming equipment. FDA and EPA have not provided clearance for this use of these material for direct contact with food.22
Sodium dichloroisocyanurate (NaDCC) has been studied by El Zawawy et al. (2010) to characterize its efficacy for inactivating the infective stages of common foodborne intestinal protozoa Entamoeba histolytica, Giardia lamblia, Cryptosporidium, Cyclospora, and the fungi Microsporidia occurring in fresh produce. They reported relative variations in susceptibility; E. histolytica and G. lamblia were most susceptible (100 percent reduction) followed by Microsporidia then Cryptosporidium and Cyclospora. Moreover, they confirmed that NaDCC did not adversely affect the consistency, color, taste, or flavor of raw green vegetables and fruits.23
In the processing of fresh fruits and vegetables, due to the resistant nature of these complex organisms, it is difficult to define and then validate chemical-aided washing as a critical control point targeting foodborne parasites.
Irradiation is an option, where regulations and consumer acceptance will allow its use. Currently in the U.S., irradiation of whole fruits and vegetables is approved only for insect control and shelf life extension, with a maximum allowable dose of 1.0 kGy. FDA has not approved the use of irradiation to enhance microbial food safety of fresh produce.
Novel Technologies
Several novel, mild, and nonthermal preservation methods have shown to be efficacious against certain foodborne parasites. High-powered ultrasound, for example, has been shown to inactivate Cryptosporidium parvum in public water supplies.
Furthermore, unpublished reports of studies by German researchers show the potential use of high-intensity pulsed electric fields (PEF) against parasites. High-intensity pulsed light (PL) and UVC light have been confirmed effective against protozoa and the larval stages of the helminths.
Pulsed light is characterized as a broad-spectrum light, wavelength 200–1,100 nm, that utilizes short, intense pulses to inactivate microorganisms. The antimicrobial activity of PL has been attributed to the short-wave UVC portion (<400 nm) of the PL spectrum and its photochemical and potentially photothermal effects. A few studies have evaluated the efficiency of PL in the inactivation of C. parvum oocysts in water and on raspberries and achieved a 2-log or greater reduction in infectivity of oocysts.24 For oocyst treatment in water samples, Lee et al. (2008)25 reported a 6-log loss in infectivity at 20 cm from the PL source after 60 s of treatment at 278 mJ/cm2. Le Goff et al. (2015)26 fed inoculated and PL-treated raspberries to mice and observed variable infection, noting an oocyst reduction of 2–3 logs using 40 mJ/cm2 PL treatment. As these studies suggest, reductions in oocyst infectivity may vary by treatment, commodity tested, and type of infectivity assay.
Pulsed light is also proposed as a postharvest treatment to inactivate protozoan parasites on leafy greens, herbs, and tomatoes, specifically C. parvum on cilantro, mesclun lettuce, spinach, and grape tomatoes. The maximum log reductions (log genomic copies) achieved on cilantro, mesclun lettuce, spinach, and tomato were 2.4, 4.3, 2.5, and 2.2, at an average dose of 0.0675 J/cm2–0.0680 J/cm2 with no significant changes in color.27
Continuous ultraviolet (UV) light at 253.7 nm using low-pressure mercury sources has been evaluated for many years as an effective disinfectant by the water industry due to the potential efficacy for controlling Cryptosporidium. Hanes et al. (2002)28 evaluated the efficacy of UV irradiation on the inactivation of C. parvum oocysts in fresh apple cider. Cider was inoculated with oocysts and exposed to 14.32 mJ/cm2 at 253.7 nm. All gamma interferon gene knockout (GKO) mice challenged with UV-treated cider demonstrated no morbidity or mortality, and infant BALB/c mice challenged with treated cider were negative for the presence of C. parvum. In contrast, the GKO mice challenged with non-UV-treated inoculated cider died, and the parasite was detected in the ileums of all challenged infant mice. This study shows that UV irradiation can be used to inactivate C. parvum in fresh apple cider.29 Also, UV demonstrated potential as a surface treatment to reduce contamination by foodborne parasites on fresh fish such as flounder. The UV dose of 46 mJ/cm2 was reported efficient against Kudoa septempunctata, a parasite found in olive flounder.30 UV merits a closer look as a single prevention measure or in combination with ozone or hydrogen peroxide washes against parasites in shellfish and raw meats.31
High hydrostatic pressure (HPP) proved to be effective in controlling these organisms in water, fresh produce, and pork products. Minimum processing pressures required are summarized in Table 1.
TABLE 1. Effectiveness of HPP in Controlling Pathogenic Parasites in Several Media (Courtesy of Vinicio Serment) 32
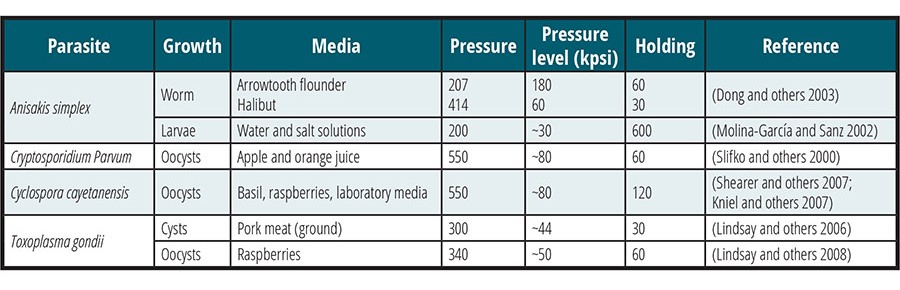
Conclusion
The soil and seas, lakes, and rivers from which we extract our food teem with life forms that can cause serious illness and suffering. Medically important parasitic helminths and protozoa are omnipresent in the natural world. They are found in farm animals, irrigation waters, manure, and on farming equipment, and they are shed by farmworkers laboring in conditions that do not give them a hygienic option for defecation. Once established in the food supply chain, these little terrors impose a big burden on public health systems. They cause illness, disease, and death. Parasites are associated with porcine-, bovine-, equine-, and ovine-derived meat and meat products. They are endemic in many fish and shellfish, as well as assorted fresh fruit and vegetables.
Foodborne parasites represent a great challenge for food safety assurance. Many medically important species are resistant to chemical treatments; others are resistant to freezing and many other go-to controls used by the food processing industry. The most successful control strategies involve a comprehensive end-to-end supply chain approach. That is, control measures are applied at any number of the high-risk nodes across the entire forward supply chain. Interventions are applied on the farm that aim to disrupt the parasite’s life cycle before it can entrench in the food supply. Measures are also applied at the point of processing and include washing, inspection, and culling defective materials. In many cases, the true critical control point for mitigating parasitic disease is at the consumer level. Cooking thoroughly is, in many instances, the most effective control measure. Cooking pork, for example, to an internal temperature greater than 160 °F (70 °C) has been shown effective for the control of Trichinella. This critical control point is managed, most often, by the end-user. The USDA’s cooking instructions attached to all meat foods are then the critical limits that define that critical control point. Likewise, cooking fish or seafood to an internal temperature greater than 140 °F (60 °C) will inactivate most seafood-borne parasites. This, too, is another case in which food safety is, in great measure, the responsibility of the consumer.
While foodborne pathogenic parasites are an important consideration for food safety plans, they are seldom identified as viable threats or as high-risk hazards on par with microbial ones. We know parasites are present in the food supply, and we also know that they are very difficult to control. This seems to be the operating principle when industry fails to identify these organisms in food safety plans: We know the parasites are there, but there is little we can do to prevent their transmission to humans.
The globalization of America’s food supply increased substantially during the 1990s and continued expanding well into the 2000s. During this interval, foodborne disease outbreaks caused by imported food appeared to rise in 2009 and again in 2010, and nearly half of the outbreaks (associated with imported food) implicated foods imported from areas that previously had not been associated with outbreaks. In that interval, food companies projected their supply chains into new and uncharted areas, where both scientific capacity and regulatory capability were absent, in search of inexpensive or exotic foods and food ingredients to keep up widening consumer tastes. Correspondingly, the risk to those same consumers of acquiring a foodborne parasite has also increased. In 1990, about 13 species of medically important parasites were of concern to food safety scientists in the U.S. Today, that figure has multiplied by more than a factor of eight.33 Recent work by researchers at the University of Washington (UW) has shown a tremendous increase of parasitic worms in the marine environment, in marine mammals, and in certain of the fish species used by humans for food.34 The cause of the increase has not been definitively set, but the UW researchers speculate that agricultural runoff and climate change may be contributing factors. The point here is that the risk is greater now for the human acquisition of medically important parasites from seafood than it has ever been. This is the type of new information that needs to be reviewed and considered by both regulatory agencies and food processors when evaluating the effectiveness of their food safety plans. Food safety is and must be a dynamic process.
Pathogenic parasites do not cause nearly as much foodborne illness in the U.S. as bacteria and viruses—CDC data estimates 2.5 million cases of foodborne illness annually due to food- and beverage-born parasites; 7 percent of the incidences, compared with 80 percent caused by viruses35—but they are still a threat to public health and the safety of the food supply system.
While the industry learns how to control parasite risk, public health officials may need to drive home food safety messaging for consuming fresh fruits and vegetables as diligently as they do for eating meat and shellfish. Consumers of these at-risk foods are, in many instances, the critical control point in preventing acquisition of a parasitic disease. Cooking, freezing, or thoroughly washing fresh fruits and vegetables before they are consumed may be the difference in whether or not one acquires a serious parasitic infection.
References
- Bruckner, D.A., 2000. “Helminthic Foodborne Infections.” Clinical Lab Med. 19: 639–660.
- https://www.foodsafetynews.com/2021/05/ireland-notes-e-coli-crypto-and-campylobacter-rises/.
- https://www.studyfinds.org/200-year-old-poop-wealthy-worms/.
- https://www.foodsafetynews.com/2021/06/toxoplasma-gondii-tops-risk-ranking-list-in-norway/.
- http://www.fao.org/publications/card/fr/c/CB1910EN/.
- https://www.cdc.gov/foodsafety/foodborne-germs.html.
- https://journals.plos.org/plosmedicine/article?id=10.1371/journal.pmed.1001923.
- https://www.who.int/news-room/fact-sheets/detail/soil-transmitted-helminth-infections.
- Nobel, E.R. and Nobel, G.A. Parasitology – The Biology of Animal Parasites, 4th edition (1976).
- http://www.fao.org/news/story/en/item/237323/icode/.
- FDA Listeria specification for ready-to-eat meat.
- https://www.fao.org/3/x5951e/x5951e01.htm.
- http://www.food-label-compliance.com/Sites/5/Downloads/Listeria-monocytogenes-Policy-Health-Canada-040111-Apr-2011.pdf.
- https://www.sciencedirect.com/science/article/pii/S095671352030517X?via=ihub.
- https://doi.org/10.1111/gcb.15048.
- Ruff, M.D. 1999. “Important Parasites in Poultry Production Systems.” Vet Parasitol. Aug 1;84(3-4): 337–47. doi: 10.1016/s0304-4017(99)00076-x. PMID: 10456422.
- CDC. 1986. “Trichinosis Surveillance.” MMWR, 35: 11SS–15SS.
- http://www.fao.org/publications/card/fr/c/CB1910EN/.
- http://www.fao.org/3/x5951e/x5951e00.htm#Contents.
- https://www.sciencedirect.com/science/article/abs/pii/S0956713504000416?via=ihub.
- https://www.ncbi.nlm.nih.gov/pmc/articles/PMC5340032/.
- https://www.cdc.gov/parasites/cyclosporiasis/outbreaks/foodborneoutbreaks.html.
- Bates, D., 2015. Personal correspondence. Cryptosporidium inactivation by high-powered ultrasound.
- https://www.semanticscholar.org/paper/Efficacy-of-Two-Peroxygen-Based-Disinfectants-for-Quílez-Sánchez-Acedo/fbacbe148eb8733adb90cd55f91b4021e409ce0a.
- https://pubmed.ncbi.nlm.nih.gov/20503596/.
- Le Goff, L., B. Hubert, L. Favennec, I. Villena, J.J. Ballet, A. Agoulon, N. Orange, and G. Gargala. 2015. “Pilot-Scale Pulsed UV Light Irradiation of Experimentally Infected Raspberries Suppresses Cryptosporidium parvum Infectivity in Immunocompetent Suckling Mice.” Journal of Food Protection, 78(12), 2247–2252. https://doi.org/10.4315/0362-028X.JFP-15-062.
- Lee, S.U., M. Joung, D.J. Yang, S.H. Park, S. Huh, W.Y. Park, and J.R. Yu. 2008. “Pulsed-UV light inactivation of Cryptosporidium parvum.” Parasitology Research, 102(6), 1293–1299. https://doi.org/10.1007/s00436-008-0908-5.
- Craighead, S., R. Huang, H. Chen, and K.E. Kniel. 2021. “The Use of Pulsed Light to Inactivate Cryptosporidium parvum Oocysts on High-Risk Commodities (Cilantro, mesclun lettuce, spinach, and tomatoes).” Food Control, Applied and Environmental Microbiology. 68(8): 4168–4172.
- Hanes, et al. 2002. “Inactivation of Cryptosporidium parvum Oocysts in Fresh Apple Cider by UV Irradiation.” Applied and Environmental Microbiology. 68(8).
- Nishioka, T., et al. 2016. “Efficacy of Sand Filtration and Ultraviolet Irradiation as Seawater Treatment to Prevent Kudoa septempunctata (Myxozoa: Multivalvulida) Infection in Olive Flounder Paralichthys olivaceus.” Fish Pathology. 51(1): 23–27. https://www.researchgate.net/publication/299416065.
- https://www.ift.org/news-and-publications/food-technology-magazine/issues/2002/april/features/parasites-and-the-food-supply.
- Serment, V. 2021. HPP white paper (Hiperbaric).
- Mead P.S., L. Slutsker, V. Dietz, L.F. McCaig, J.S. Bresee, C. Shapiro, P.M. Griffin, R.V. Tauxe. 1999. “Food-Related Illness and Death in the United States.” Emerg Infect Dis. Sep–Oct;5(5): 607–625. doi: 10.3201/eid0505.990502. PMID: 10511517; PMCID: PMC2627714.
- https://www.washington.edu/news/2020/03/19/sushi-parasites-have-increased-283-fold-in-past-40-years/.
- https://www.cdc.gov/foodborneburden/2011-foodborne-estimates.html.
Larry Keener, CFS, PA, PCQI, is President and CEO of International Product Safety Consultants. He is also a member of the editorial advisory board of Food Safety Magazine.
Tatiana Koutchma, Ph.D., is a Research Scientist at Agriculture and Agri-Food Canada specializing in novel food processing technologies.