Monitoring aw is a critical control point for many food industry operations.
The importance of water activity (aw) in food systems cannot be overemphasized. Throughout history water activity in food has been controlled by drying, addition of sugar or salt and freezing. These methods prevent spoilage and maintain food quality. Water activity is the ratio of the partial vapor pressure of water in equilibrium with a food to the partial saturation vapor pressure of water vapor in air at the same temperature. This is equal to the relative humidity of air in equilibrium with the food. The water activity of a food describes the energy state of water in the food, and hence its potential to act as a solvent and participate in chemical/biochemical reactions and growth of microorganisms. It is an important property that is used to predict the stability and safety of food with respect to microbial growth, rates of deteriorative reactions and chemical/physical properties.
The growing recognition of the water activity principle is illustrated by its incorporation into U.S. Food and Drug Administration (FDA) and U.S. Department of Agriculture (USDA) regulations, Good Manufacturing Practices (GMP) and Hazard Analysis & Critical Control Points (HACCP) requirements, and most recently in NSF International Draft Standard 75. The purpose of these regulations is to detail the specific requirements, critical control points and practices to be followed by industry to assure that products are produced under sanitary conditions and are pure, wholesome and safe. New instrument technologies have vastly improved speed, accuracy and reliability of water activity measurements and are definitely a needed tool for food safety and quality.
Background
Throughout history man has controlled the water activity of food through drying, addition of salt or sugar and freezing such that the food becomes stable to microbial and chemical deterioration. Food manufacturers today have the same goal of making a stable and safe product. This means that the products must be wholesome and not endanger the health of the consumer with microorganisms or their toxins. The advantage today is in the knowledge and understanding of the importance of water activity in controlling microbial growth and thus upon the shelf life and safety of a product.
The Centers for Disease Control (CDC) stated foodborne disease is responsible for approximately 76 million illnesses, 325,000 hospitalizations and 5,000 deaths annually in the United States. Known pathogens, such as Salmonella, Escherichia coli O157:H7, Campylobacter and Listeria monocytogenes, account for an estimated 14 million illnesses, 60,000 hospitalizations and 1,800 deaths alone. With staggering statistics like these one would not believe that the U.S. has the safest food supply. Based on FoodNet surveillance data from 1997 to 1999, illness from the most common bacterial foodborne pathogens declined nearly 20%. This decline represents at least 855,000 fewer Americans each year suffering from foodborne illness caused by bacteria since 1997. By using water activity and controlling major food risks, such as microbial contaminants, the food industry can better ensure the safety of its products.
The consequence of a microbiological failure, particularly as they relate to product recalls, can be very costly. Brand recognition and sales may ultimately suffer as a result of consumers relating the recall to other products manufactured by a particular company. In a world of increasing pressures and diminishing resources, the need to strengthen microbiological quality assurance programs has not abated. In fact, there is more pressure than ever on the management of microbiological quality. Food safety must be controlled during the production process from beginning to end, rather than relying on detection of problems in the finished product.
Very few intrinsic properties are as important as water activity in predicting the survival of microorganisms in a food product. Scott showed that microorganisms have a limiting water activity level below which they will not grow.[1] Water activity, not water content, determines the lower limit of available water for microbial growth. The lowest aw at which the vast majority of food spoilage bacteria will grow is about 0.90. Staphylococcus aureus under anaerobic conditions is inhibited at an aw of 0.91, but aerobically the a level is 0.86. The aw for mold and yeast growth is about 0.61 with the lower limit for growth of mycotoxigenic molds at 0.78 aw.[2] Table 1 lists the water activity limits for growth of microorganisms significant to public health and examples of foods in those ranges.
Water Activity Defined
Water activity is derived from fundamental principles of thermodynamics and physical chemistry. As a thermodynamic principle there are requirement in defining water activity that must be met. These requirements are: pure water (aw = 1.0) is the standard state, the system is in equilibrium, and the temperature is defined. In the equilibrium state: µ=µo + RT 1n (f/fo)
where: µ (J mol-1) is the chemical potential of the system i.e. thermodynamic activity or energy per mole of substance; is the chemical potential of the pure material at the temperature T (°K); R is the gas constant (8.314 J mol-1 K-1); f is the fugacity or the escaping tendency of a substance; and fo is escaping tendency of pure material.[3] The activity of a species is defined as a = f/fo. When dealing with water, a subscript is designated for the substance, aw = f/fo
where aw is activity of water, or the escaping tendency of water in system divided by the escaping tendency of pure water with no radius of curvature. For practical purposes, under most conditions in which foods are found, the fugacity is closely approximated by the vapor pressure (f ≈ p) so that: aw = f/fo ≈ p/po
Equilibrium is obtained in a system when µ is the same everywhere in the system. Equilibrium between the liquid and the vapor phases implies that µ is the same in both phases. It is this fact that allows the measurement of the vapor phase to determine the water activity of the sample.
Water activity is defined as the ratio of the vapor pressure of water in a material (p) to the vapor pressure of pure water (po) at the same temperature. Relative humidity of air is defined as the ratio of the vapor pressure of air to its saturation vapor pressure. When vapor and temperature equilibrium are obtained, the water activity of the sample is equal to the relative humidity of air surrounding the sample in a sealed measurement chamber. Multiplication of water activity by 100 gives the equilibrium relative humidity (ERR) in percent. aw = p/po = ERH (u/0)/100
Water activity is a measure of the energy status of the water in a system. There are several factors that control water activity in a system. Colligative effects of dissolved species (e.g. salt or sugar) interact with water through dipole-dipole, ionic and hydrogen bonds. Capillary effect where the vapor pressure of water above a curved liquid meniscus is less than that of pure water because of changes in the hydrogen bonding between water molecules. Surface interactions in which water interacts directly with chemical groups on undissolved ingredients (e.g. starches and proteins) through dipole-dipole forces, ionic bonds (H3O or OH-), van der Waals forces (hydrophobic bonds), and hydrogen bonds. It is a combination of these three factors in a food product that reduces the energy of the water and thus reduces the relative humidity as compared to pure water. These factors can be grouped under two broad categories osmotic and matric effects.
Due to varying degrees of osmotic and matric interactions, water activity describes the continuum of energy states of the water in a system. The water appears ‘bound” by forces to varying degrees. This is a continuum of energy states rather than a static “boundness.” Water activity is sometimes defined as “free,” “bound,” or “available water” in a system. Although these terms are easier to conceptualize, they fail to adequately define all aspects of the concept of water activity.
Water activity is temperature dependent. Temperature changes water activity due to changes in water binding, dissociation of water, solubility of so lutes in water, or the state of the matrix. Although solubility of solutes can be a controlling factor, control is usually from the state of the matrix. Since the state of the matrix (glassy vs. rubbery state) is dependent on temperature, one should not be surprised that temperature affects the water activity of the food. The effect of temperature on the water activity of a food is product specific. Some products increase water activity with increasing temperature, others decrease aw with increasing temperature, while most high-moisture foods have negligible change with temperature. One cannot, therefore, predict even the direction of the change of water activity with temperature, since it depends on how temperature affects the factors that control water activity in the food.
As a potential energy measurement it is a driving force for water movement from regions of high water activity to regions of low water activity. Examples of this dynamic property of water activity are include moisture migration in multidomain foods (e.g. cracker-cheese sandwich), the movement of water from soil to the leaves of plants, and cell turgor pressure. Since microbial cells are high concentrations of solute surrounded by semipermeable membranes, the osmotic effect on the free energy of the water is important for determining microbial water relations and therefore their growth rates.
Advances in the Measurement of Water Activity
There is no device that can be put into a food that directly measures the water activity. Rather, a is measured with an indirect method as described above. A food sample is placed in a small chamber and the water in the air is measured after it equilibrates with the sample. Methods for water activity determinations are detailed in the Official Methods of Analysis of AOAC International.[4] New instrument technologies have vastly improved speed, accuracy and reliability of measurements. Reliable laboratory instrumentation is required to guarantee the safety of food products and enforce government regulations. Two different types of water activity instruments are commercially available. One uses chilled mirror dew- point technology while the other measures relative humidity with sensors that change electrical resistance or capacitance. Each has advantages and disadvantages. The methods vary in accuracy, repeatability, speed of measurement, stability in calibration, linearity and convenience of use.
Application of the Water Activity Concept
From the previous definition, it is easily understood how water activity is useful in predicting food safety and stability with respect to microbial growth, chemical/biochemical reaction rates and physical properties. By measuring and controlling the water activity of foodstuffs, it is possible to predict which microorganisms will be potential sources of spoilage and infection; maintain the chemical stability of foods; minimize nonenzymatic browning reactions and spontaneous autocatalytic lipid oxidation reactions; prolong the activity of enzymes and vitamins in food; and optimize the physical properties of foods, such as texture and shelf life.[5,6] A global stability map (Figure 1) of foods shows these factors as a function of water activity.[7]
The growing recognition of the water activity principle is illustrated by its incorporation into FDA and USDA regulations, GMP and HACCP requirements, and most recently in NSF International Draft Standard 75. Water activity is an important critical control point for risk analysis as defined by the HACCP concept. These regulations and requirements are based on the current FDA Food Code definition of potentially hazardous foods. Potentially hazardous foods are those that require temperature control because they support the rapid and progressive growth of pathogenic microorganisms. Potentially hazardous food does not include items with water activity values of 0.85 or less, food with a pH level of 4.6 or less, food in unopened hermetically sealed containers, those that maintain commercial sterility under nonrefrigerated storage and distribution, or those in which rapid and progressive growth of pathogenic microorganisms cannot occur.
Conclusion
Prevention will continue to be central to food safety from both the processor and government perspective. Many companies are already using HACCP voluntarily, in addition to the mandatory meat, poultry and seafood programs. Included in GMP and HACCP programs are steps requiring early warning system, risk assessment, improved detection/control methods and improved inspections and compliance.
Monitoring water activity is a critical control point for many manufacturers and should be incorporated into many other food safety programs. The number one priority is protecting the consumer. Recalls can cost millions of dollars in product losses and operational delays, along with losses to consumer confidence and a company’s reputation. Incorporating water activity testing and other science based analyses into a food safety program helps ensure the highest quality and safest food supply.
Anthony J. Fontana Jr., Ph.D., is an applications engineer at Decagon Devices, Inc. He provides the scientific and technical customer support, and makes presentations of products, technologies, techniques and applications to customers. He also assists in product/technology development and testing. Prior to his work at Decagon, he managed a quality control laboratory for a large cheese, whey protein concentrated, alcohol and animal feed mix manufacturer. Fontana received his bachelor degree in biochemistry from University of California, Riverside in 1985, and his doctorate degree in agricultural and environmental chemistry from University of California, Davis in 1994.
Presented at the Second NSF International Conference on Food Safety, October 2000, Savannah, GA.
References
1. Scott, W.J. Water relations of food spoilage microorganisms. Advances in Food Research (7) pp. 83-1 27. 1957.
2. Beuchat, L.R. Microbial stability as affected by water activity. Cereal Foods World, 26(7), pp. 345-349. 1981.
3. van den Berg, C. and S. Bruin. Water activity and its estimation in food systems: theoretical aspects. In: Water Activity: Influences on Food Quality. LB. Rockland and G.E. Stewart, eds. Academic Press, New York, pp.1-61. 1981.
4. AQAC International. Official Methods of Analysis of AOAC International. T.R. Mulvaney, ed. AQAC International. Arlington, VA, pp.42-i- 42-2. 1995.
5. Fontana, A.J. Water activity: why it is important for food safety. Proceedings of the First NSF International Conference on Food Safety. Albuquerque, NM, pp.177-185. 1998.
6. Fontana, A.J. Understanding the importance of water activity in food. Cereal Foods World 45(1), pp. 7-10. 2000.
7. Labuza, T.P. Properties of water as related to the keeping quality of foods. Proceedings of the Third International Congress of Food Science & Technology. Washington, DC., pp. 618-635. 1970.
8. Beuchat, L.R. Influence of water activity on growth, metabolic activities, and survival of yeasts and molds. Journal of Food Protection (46), pp. 135-141. 1983.
Water Activity’s Role in Food Safety and Quality
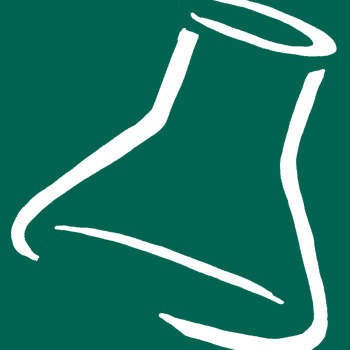