Given the non-biodegradability, mobility, and persistence of metals in the environment, metals can enter into food. Consequently, testing for metals in foods and food additives, such as colorants, is an important focus area for AOAC INTERNATIONAL (AOAC). The AOAC's metals scientific community has formed a working group to review various regulations and new requirements for trace metals content in food and colorants with the intent to update the current Standard Method Performance Requirements (SMPR®) for a selection of metals.
This work shall set the framework for the development of Official Methods of AnalysisSM (OMA), which are tested to meet the performance requirements outlined in the SMPR. Since AOAC is an international organization with a focus on global methods and performance harmonization, the working group also reviews existing methods based on Codex Alimentarius Commission (Codex) requirements for metals analysis in various food commodities. In this article, the authors discuss the potential sources and challenges associated with metals in food, recent development in international regulatory limits, the SMPR revision, and relevant activities related to Codex.
Background on Metals Toxicity and Testing
While various factors can influence the safety of foods and food additives, including microbial, pesticide, and other residues, one that is particularly challenging to address (and often under-represented in the food industry) is that of elemental contaminants—commonly referred to as metals. To understand the safety concerns related to metals, it is important to first understand that not all elements are harmful to human health. On the contrary, many elements, both at high concentrations (macro) and low concentrations (micro), are essential to life. As an example, elements such as sodium (Na), potassium (K), calcium (Ca), and magnesium (Mg) are essential macronutrients, being drivers for a number of crucial functions within the body, whereas others such as copper (Cu), manganese (Mn), and selenium (Se) are essential micronutrients. Furthermore, there are other elements that appear to have no biological function at all, and may elicit a toxicological response depending on various factors. This last class of elements, which comprises metals and metalloids, is the focus of this article. However, it is important to note that anything has the potential to act as a toxicant; what determines its toxicity is ultimately its concentration. Going forward, for the sake of simplicity, these toxic metals and metalloids shall be collectively referred to as "metals."
The question of what makes elemental contaminants so pervasive relates to their source. Metals are ubiquitous in the environment, being found throughout the continental crusts at varying concentrations and forms, depending upon their location. While all metals exist at some natural bioavailable concentration in the environment as a consequence of natural processes such as weathering, volcanic activity, sea spray, and so on, the rate of their release into the environment can also be increased as a result of mining, industrialization, and other anthropogenic activities.
Unlike other classes of pollutants, such as persistent organic pollutants (POPs), metals cannot be broken down into simpler forms and are persistent in the environment. What ultimately determines the toxicity of a metal is the type of metal, its concentration, and its chemical form, where the latter determines its mobility and availability for uptake by plants and organisms. For example, arsenic is a known toxic element; however, it is present in the environment in different chemical forms with varying degrees of toxicity. Arsenobetaine, a methylated form of arsenic, is regarded as being relatively nontoxic and is the most common form of arsenic found in seafoods. Its inorganic counterparts, in contrast, tend to be regarded as significantly more toxic at the same concentration levels.
As discussed, metals can make their way into the environment via various means and result in the contamination of air, water, and soil. Plants grown in fields with high concentrations of metals in soil and water will likely be observed to have significant concentrations of those metals, with different concentrations in different parts of the plants, depending on the plant species.1
For monitoring of metals, a few common techniques can be employed; these will be discussed later in this article. The analysis of metals can be separated into two major tracks, namely those that examine the total elemental concentration and those that examine the chemical species of the metal (also known as speciation analysis). The elemental analysis of metals is the simpler of the two. Foods and food additives are digested in acid (or a combination of acids), which keeps the metals in suspension, and this digested sample is then introduced to an elemental analysis technique.
There are a few elemental analysis techniques, in order of decreasing detection limit, that have historically been used for the screening of metals:
- Flame Atomic Absorption Spectroscopy (FAAS, also known as AAS), which evaluates the light absorbed by a metal during excitation in a flame
- Graphite Furnace Atomic Absorption Spectroscopy (GFAAS), which is a variant of FAAS with lower detection limits
- Inductively Coupled Plasma Optical Emission Spectrometry (ICP-OES), which evaluates the light emitted by elements and ions as they return to their ground state following excitation in an argon plasma
- Inductively Coupled Plasma Mass Spectrometry (ICP-MS), which assesses the concentrations of metals in a sample based upon the mass-to-charge ratio that is characteristic for that particular element following ionization in an argon plasma.
While many of these techniques have been used throughout history, ICP-MS has become the instrument of choice for trace metals analyses due to its sensitivity and the low concentrations at which many metals elicit a toxic response. The other techniques typically used for the second-track (speciation) of analyses of metals are hyphenated techniques with ICP-MS, namely High-Performance Liquid Chromatography ICP-MS (HPLC-ICP-MS) and Gas Chromatography ICP-MS (GC-ICP-MS). These techniques, while extremely informative, can be more challenging to execute well since it is necessary to ensure that the chemical forms of the metals do not change during sample collection, storage, and processing.
Typically, with metals analyses of foods and food additives, the concentrations measured are compared against local and regional standards. Given the global nature of the food trade, it would be advantageous to establish and use common standards and methods. This would ensure that results are comparable across regions, and that a commodity that is accepted in terms of metals content for its country of provenance is not rejected in another. It is here that organizations such as AOAC INTERNATIONAL become important.
AOAC INTERNATIONAL
AOAC became established in 1884 within the U.S. Department of Agriculture (USDA) Bureau of Chemistry. Some 140 years later, AOAC INTERNATIONAL is an independent, nonprofit, professional organization still pushing the limits of existing standards, and has expanded beyond the agricultural sciences to include various aspects of food safety, authenticity, and provenance. Staying true to the cause, AOAC's mission is to "Advance food safety and product integrity through standards, validated test methods, and laboratory quality programs." Within the organization, the core programs and services of AOAC work together to ensure that quality control and assurance metrics are in place in labs worldwide.
At present, many labs around the world use different testing methods, protocols, and standards, which can lead to inconsistent results with questionable data quality and integrity. To tackle this problem, AOAC works with global leaders to develop aligned standards, the Official Methods of AnalysisSM (OMA). This process is initiated via the creation of an advisory panel of key opinion leaders who identify key stakeholders, frame the issues, and set priorities. The advisory panel assignment is followed by the development of professional working groups to characterize the current methods and standards landscape, address areas of contention including target issues raised by the advisory panel, and identify potential solutions and improvements.
Some of these target issues may include fitness for purpose, stability, interferences, sample extraction, limits of detection, and quality control metrics. The main output of the working group is a Standard Method Performance Requirement (SMPR) document that is used in a public call for methods to address the issues shared by the advisory panel. The method performance requirements are short documents that include background information, the scope of the method (applicability), validation guidance, and other areas considered critical to the method performance success. Public comments on these method performance requirements are then considered and reconciled. Final suggestions are made to the stakeholder panel, which comprises representatives from government, industry, and academic sectors worldwide.
The next step involves an Expert Review Panel (ERP), whose volunteer members meticulously scrutinize and review the methods submitted to AOAC that are intended to fulfill the method performance requirements outlined in the SMPR document. The ERPs consist of key stakeholders who are expected to work collectively toward meaningful, balanced, and unbiased results. The Official Methods Board makes the decision regarding the Final Action status of methods to be published in the OMA.
Metals Community Activities
One of the specialized groups within AOAC is the metals community, which is led by co-chairs Kevin Kubachka, Ph.D. and Eve Kroukamp, Ph.D., and comprises people from various backgrounds who are interested in metals analysis. The group meets throughout the year, with one primary meeting at the AOAC Annual Meeting and Exposition to discuss current and emerging trends in food and agriculture, as well as to identify unmet needs and risks. The outcomes from these meetings can be used to establish working groups and help direct the AOAC's efforts in establishing new methodology. For example, a few years ago, many community members expressed interest in a unified method for metals determination in hemp and cannabis. The group discussed the challenges, needs, current methods, and regulations, and recommended that the AOAC focus efforts in this area. This led to working groups, SMPRs, and a candidate method recently approved for First Action Official Methods status.
Within the AOAC metals community, there is a great deal of knowledge and expertise in metals analyses, and members from this group have often been solicited for their participation in working groups and expert review panels to assist the AOAC when expertise related to elemental analysis is needed. The group maintains communication using an email list and a LinkedIn group page. These platforms are used to alert members of events, pose questions, drive engagement, and solicit expertise. One of the many focus areas of the AOAC Metals Working Group is that of metals in food, baby foods, and food colorants.
Metals in Baby Foods—Recent Concerns
The past decade has seen several media reports discussing the presence of elemental contaminants—specifically arsenic, cadmium, lead, and mercury—in foods marketed toward infants and young children. This population subset is at an increased risk due to factors such as their smaller body size and developing organs, which can impact their ability to metabolize and detoxify metals and other toxicants. In 2021, a U.S. bill was introduced seeking safety requirements related to inorganic arsenic, cadmium, lead, and mercury in infant and toddler foods.2 This legislation, titled The Baby Food Safety Act of 2021, called for limits of the aforementioned elements in foods marketed to children under 36 months (referred herein as baby food) that are markedly lower than previously established limits. The U.S. Food and Drug Administration (FDA) has established the Closer to Zero Action Plan3 in an effort to ensure that food manufacturers take the steps necessary to reduce exposure of infants and young children to those contaminants.
When examining the reported findings of the various studies and publications within the scientific literature, there are several general trends related to arsenic, cadmium, lead, and mercury in baby food and its components. For example, levels of lead and cadmium in root vegetables (carrots, sweet potatoes, etc.) are generally higher compared to other foods, often due to the direct contact of the edible portion of the plant with soil and the inability of the plant to translocate these metals to non-edible portions. Leafy greens, such as kale, may accumulate specific metals at high concentrations, and so these plants, when grown in cadmium-containing soils, are often found to contain significant levels of cadmium. Also, it is well established that rice can accumulate significant levels of arsenic (and subsequently inorganic arsenic) from the flooded crops.
While seafood generally contains high levels of arsenic, nontoxic forms generally predominate. In contrast, mercury in seafood typically tends to be in the form of methyl mercury due to the natural methylation of mercury by anerobic bacteria present in these environments. Mercury is also known to biomagnify, and due to its lipophilic properties it deposits into fatty tissues, which is why this metal is often associated with piscivorous fish with high fat content in their muscle tissue, such as tuna.
While foods containing no toxic elements would be ideal, this is essentially unachievable due to their ubiquity in the environment. The presence of arsenic, cadmium, lead, and mercury often derives from various environmental and processing factors. Although there may be ways to reduce the presence of metals in foods at the source, quality control via analysis ensures that contaminated foods do not enter the market.
FDA's Closer to Zero action plan is a continual process that includes the review of scientific data related to metals contamination in foods, toxicity studies, and analytical advancements, among others, to help establish appropriate limits for these contaminants. As regulatory limits become lower, accurate and reliable methods are needed to analyze these metals contaminants at concentrations below these limits to ensure the data confidence needed to enforce the regulations. For example, a regulatory limit of 10 parts per billion (ppb or µg/kg) for a particular element in a commodity would require the method's limit of quantitation (LOQ) to be significantly lower, approximately 2–5 ppb (generally, LOQ should be ½ to ¼ of the regulatory limit).
Detection of arsenic, cadmium, lead, and mercury at low concentrations (< 5 ppb) in a food matrix can be quite challenging from an analytical perspective and requires suitable guidance in terms of the instrumentation used and methodological approaches. As previously described, an AOAC Metals Working Group has been assigned to help establish an SMPR, which ensures that prospective methods are developed so that appropriate quality controls are in place. This document will be used to guide methods that will help enforce regulations and provide reliable data for food safety assessments.
Several countries have established regulatory limits for arsenic (inorganic arsenic), cadmium, lead, and mercury in a wide range of food commodities. A few are mentioned in the following text and are intended to be provided simply as examples, as these limits are constantly changing. In 2020, FDA issued final guidance for industry that included an action level of 100 ppb of inorganic arsenic in infant rice cereal.4 Additionally, FDA has recently issued final guidance for industry with an action level of 10 ppb inorganic arsenic in apple juice.5 At the time of preparation of this manuscript, draft guidances for allowable lead concentrations in foods intended for babies and young children have set action levels at 10 ppb for fruits, vegetables (excluding single-ingredient root vegetables), mixtures, yogurts, custards/puddings, and single-ingredient meats; and at 20 ppb for root vegetables (single ingredient) and dry infant cereals.6 In contrast, the EU has set maximum levels of 20 ppb of inorganic arsenic in baby foods, fruit juices, and infant formula marketed as powders, as well as a limit of 10 ppb inorganic arsenic for infant formula marketed as a liquid.7 China has also set limits for several commodities including a limit of 100 ppb of inorganic arsenic in canned foods for infants and young children and 200 ppb for cereal-based foods for infants and young children.8
Codex Alimentarius Commission
The Codex Alimentarius Commission is part of the World Health Organization (WHO) and is the foods standards setting body. Codex standards are used worldwide to harmonize national food safety regulations. Codex is recognized in the World Trade Organization (WTO) Agreement on the Application of Sanitary and Phytosanitary (SPS) Measures as the international reference point for food safety. One Codex standard (CXS 234-1999) includes recommended methods of analysis and sampling to verify provisions in Codex standards.
Countries and global standards-developing organizations such as AOAC INTERNATIONAL can submit proposals for the adoption of analytical methods meeting Codex requirements. In 2022, the Codex Committee on Contaminants in Foods (CCCF) agreed to review the analytical methods for elemental contaminants in Codex standards under its responsibility for possible inclusion in CXS 234-1999. CCCF proposed method performance criteria for lead and cadmium, based on maximum limits for these analytes in commodities. These limits were endorsed by the Codex Committee on Methods of Analysis and Sampling (CCMAS) and adopted by the Codex Alimentarius Commission (REP23MAS, REP23CAC), respectively (e.g. limits of quantification for lead at 4 ppb in infant formula and ranging from 8–20 ppb in other specified food commodities).
Standards Development
With the above perspectives for metals in food and new regulatory proposals, AOAC convened an Advisory Panel in January 2023, which issued guidance to establish a working group to begin the process to produce an Official Method of Analysis to meet the new challenges for metal contaminants in foods, including foods developed for infants and young children. The Advisory Panel members include food and beverage manufacturers, equipment manufacturers, and the Infant Nutrition Council of America. With support from the Advisory Panel, a working group was formed to create an SMPR for the metals community.
The working group is co-chaired by Stéphane Dubascoux of Nestlé and Christopher Smith of the Coca-Cola Company. This group combined resources with the AOAC Color Additives from Natural Sources Working Group to ensure that the SMPR developed can also be used in the characterization of common colorants employed within the food industry. The Advisory Panel's role is to organize the Metals Working Group, which comprises a diverse group of volunteers from industry, academia, national and regional governments, equipment manufacturers, and others. This group met to create consensus requirements for candidate methods and discuss the analytical science needed to meet these requirements. The SMPR document outlines the requirements that the metals community believes are necessary to aid the development of an official method of appropriate robustness and quality.
Given the wide range of possible metal contaminants, the scope and applicability for the SMPR were narrowed to the four compulsory toxic metals—arsenic, cadmium, lead, and mercury—while allowing additional optional metals to be evaluated as part of the proposed method. Matrices of emphasis were food (and beverages), baby foods, and food additives, including colorants. Throughout 2023, the Metals Working Group met virtually, and a first draft SMPR was released in June 2023 for review. The draft is currently pending finalization. One of the discussion points for the working group was related to which analytical technique should be used. The group determined that ICP-MS was the analytical technique of choice, given the low concentrations of the metals under scrutiny.
As previously mentioned, ICP-MS is recognized to be the most sensitive analytical tool for the routine analysis of trace elements (including toxic metals) in various matrices, including food. Despite the ultra-low detection limits of this technique, the existing official international methodologies/standards implementing ICP-MS (EN15763:2009; EN17851:2023; FDA E.A.M 4.7), have LOQs that are not amenable to the above-mentioned proposed or active regulatory limits from various countries. Meeting these limits is a substantial analytical challenge and requires highly optimized methods, instrumentation, and knowledge. Table 1 summarizes the limits of quantification of these existing standards for arsenic, cadmium, lead, and mercury. To address the challenges of these new regulatory limits, the group proposed the SMPR for Determination of Trace Elemental Contaminants in Food and Beverages, presented in Table 2, to ensure that the proposed methods will meet the anticipated needs of the community.

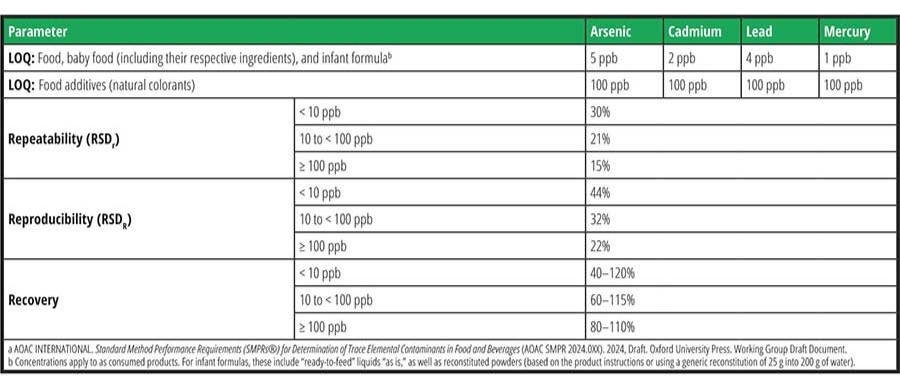
Despite the analytical challenges encountered in elemental analysis, the current level of maturity of ICP-MS should allow for the achievement of these targets. To further improve method detection limits and ensure that the results are accurate and reflective of the true levels, labs would benefit from reducing sources of contamination from collection through to analysis. Moreover, careful quality control will be needed to ensure measurement accuracy—for example, the use of well-characterized reference materials.
Takeaway
In conclusion, while still largely underrepresented in food safety standards, there has been increased awareness of the contamination of foods by metals over the past decade. To address these concerns, regulatory bodies are continuing to evaluate, establish, and adjust safety limits for trace metals in a variety of food commodities as knowledge related to source, mobility, dose, uptake, and response increase.
For the accurate characterization of these metals at trace concentrations, methodological improvements are critical. To aid this, organizations such as AOAC INTERNATIONAL, the Codex Alimentarius Commission, and other international groups bring various stakeholders together, ranging from regional regulatory bodies, food manufacturers, and analytical instrument manufacturers to testing laboratories. This ensures better alignment, visibility, and quality control in the food and agricultural markets.
Note
The opinions stated herein are those of the authors, and do not reflect the views of their employers.
References
- Abedi, T., and A. Mojiri. "Arsenic Uptake and Accumulation Mechanisms in Rice Species" Plants 9, no. 2 (2020): 129. https://doi.org/10.3390/plants9020129.
- 117th U.S. Congress. Baby Food Safety Act of 2021. March 29, 2021. https://www.congress.gov/bill/117th-congress/house-bill/2229.
- U.S. Food and Drug Administration (FDA). "Closer to Zero: Reducing Childhood Exposure to Contaminants from Food." February 5, 2024. https://www.fda.gov/food/environmental-contaminants-food/closer-zero-reducing-childhood-exposure-contaminants-foods.
- FDA. Guidance for Industry: Action Level for Inorganic Arsenic in Rice Cereals for Infants. August 2020. https://www.fda.gov/regulatory-information/search-fda-guidance-documents/guidance-industry-action-level-inorganic-arsenic-rice-cereals-infants.
- FDA. Guidance for Industry: Action Level for Inorganic Arsenic in Apple Juice. June 2023. https://www.fda.gov/regulatory-information/search-fda-guidance-documents/guidance-industry-action-level-inorganic-arsenic-apple-juice.
- FDA. Draft Guidance for Industry: Action Levels for Lead in Food Intended for Babies and Young Children. January 2023. https://www.fda.gov/regulatory-information/search-fda-guidance-documents/draft-guidance-industry-action-levels-lead-food-intended-babies-and-young-children.
- European Commission. "Commission Regulation (EU) 2023/465: Amending Regulation (EC) No 1881/2006 as regards maximum levels of arsenic in certain foods." European Union. March 3, 2023. https://eur-lex.europa.eu/legal-content/EN/TXT/HTML/?uri=CELEX:32023R0465#d1e32-54-1.
- USDA Foreign Agricultural Service. "China Releases the Standard for Maximum Levels of Contaminants in Foods." FAS Staff, Beijing, China. May 9, 2018. https://apps.fas.usda.gov/newgainapi/api/report/downloadreportbyfilename?filename=China%20Releases%20the%20Standard%20for%20Levels%20of%20Contaminants%20in%20Foods%20_Beijing_China%20-%20Peoples%20Republic%20of_5-9-2018.pdf.
Eve Kroukamp, Ph.D., is the AOAC Metals Community Co-Chair and a member of the AOAC INTERNATIONAL Metals Expert Review Panel.
Christopher J. Smith, Ph.D., is the AOAC INTERNATIONAL Metals Working Group Co-Chair and the Senior Director of Flavor Supply for the Coca-Cola Company.
Kevin Kubachka, Ph.D., is the AOAC Metals Community Co-Chair and the AOAC INTERNATIONAL Metals Expert Review Panel Chair, as well as an Analytical Chemist at the U.S. Food and Drug Administration.
Stéphane Dubascoux, Ph.D., is the AOAC INTERNATIONAL Metals Working Group Co-Chair and an R&D Senior Specialist and Group Leader for the Minerals and Metals Team at the Nestlé Institute of Food Safety and Analytical Sciences, part of Nestlé Research at Société des Produits Nestlé S.A
Erik Konings, Ph.D., is an R&D Expert at the Nestlé Institute of Food Safety and Analytical Sciences, part of Nestlé Research at Société des Produits Nestlé S.A.