To the average consumer, food packaging is simply the enclosure of a food product in a plastic pouch, a metal can or a glass bottle. To a scientist or engineer, however, food packaging is a rather technical matter. It is a coordinated system designed for the efficient delivery of high-quality, safe food products throughout every phase of the supply chain.[1]
Primary packaging has a great influence on food quality and safety. It can be considered a four-component system: the food, the internal environment, the package and the external environment. A good understanding of the interactions between these components is necessary to design the food package. Interactions between the food and the internal environment are the most important factors, since the rate of food deterioration under the conditions of the internal environment frequently governs the shelf life of the package. The package represents a protection of the food against negative effects from the external environment. It often serves as a barrier to delay the ingress of moisture or oxygen from the external environment, thereby extending the shelf life of foods that are sensitive to moisture and oxygen. Interactions between food and package may be desirable or not. Desirable interactions include active packaging systems that will be described in detail below, whereas undesirable interactions include migration of undesirable packaging components to the food.[1]
In recent years, several technologies have received great attention due to their innovative and challenging characteristics, such as: 1) active packaging, 2) bio-based/edible materials, 3) intelligent packaging and 4) high-barrier materials. We give here an overview of the general concepts regarding active packaging systems, edible coatings and films, and we present several recent research advancements that could drive the transition toward more efficient and innovative systems based on the use of novel concepts of macromolecular design. The control of hierarchical structures from molecular levels to nano- and microscale through the control of the interactions and assembling behavior of molecules and compounds will permit development of materials with advanced and smart functionalities as well as materials able to interact with and respond to external stimuli, thus improving the efficiency of the packaging system.
Active Packaging
Active packaging has been defined as systems in which the product, the package and the environment interact in a positive way to extend the food’s shelf life or to achieve some characteristic of the food that cannot be obtained otherwise. Active systems are usually obtained by embedding into the packaging materials active compounds that absorb substances from the environment surrounding the food or that are released from the packaging into the food. Among the several active systems, interesting and challenging materials are those able to release active compounds such as antimicrobials or antioxidants into the food. In this respect, the aim of controlled-release systems intended for food packaging applications is to optimize the amount of active compounds released from the polymeric carrier into the food to delay or inhibit the mechanisms responsible for the degradation of the packed foodstuff.
EU Regulations 1935/2004 and 450/2009/EC define specific rules for the use of new types of materials and articles designed to actively maintain or improve the condition of the food. In particular, Regulation 1935/2004 offered for the first time the opportunity to use active packaging in Europe by allowing the application of materials with agents that could migrate into foods. This regulation regarding all materials and articles intended to come into contact with food contains general provisions on the safety of active and intelligent packaging and sets the framework for the European Food Safety Agency evaluation process.[2]
Currently, one of the most interesting approaches to improve the performance of active packaging systems is the development, on a laboratory scale, of hybrid organic-inorganic materials. Two different approaches can be used: 1) systems in which the active inorganic particles are dispersed at the nanometric level into the polymer matrix, and 2) systems in which the inorganic particles are embedded into the polymer matrix and act as a carrier that enables the controlled release of the active compound from the packaging into the food.
An example of the first approach is given by inorganic phyllosilicate clays that can be used as support for silver nanoparticles (AgNPs) to generate a new class of antimicrobial systems. The platelet clays, which possess intensive charges on their surfaces, swell in water and generate a stable pseudo-cross-linking network that interacts and stabilizes the AgNPs. The silver-supporting material can control the release kinetics of silver ions because of weak electrostatic interactions, which are established with surface platelets of montmorillonite (MMT). We have recently shown that silver-MMT (Ag-MMT) antimicrobial nanoparticles can be obtained by incorporating silver ions into natural MMT.[3] These Ag-MMT nanoparticles can be embedded in bio-based polymer matrices to explicate antimicrobial activities against microorganisms. It was shown that water uptake by the organic matrix plays a key role in the antimicrobial efficacy of the developed active films. Agar hydrogel nanocomposites, which exhibit the highest water content uptake, were the most active against the tested microorganisms.
An example of the second approach is given by highly ordered mesoporous silicas that have been long recognized as very promising materials with a wide range of possible applications, such as the capability to act as a reservoir for the accommodation of drug molecules in the pharmaceutical field or active compounds in the food packaging field.[4] In fact, the well-known possibility of chemically functionalizing the pore surface of siliceous mesostructures with different organic moieties[5] constitutes a route for controlling drug release by diffusion under specific conditions. When the interactions between desorbing molecules and silica pore walls are significantly strong and/or show some kind of specificity, the release also depends on the stability of the complex between the functional groups of the drug/active compound and those of the substrate. This phenomenon permits the fine-tuning of the release of specific molecules from a given mesostructure by simply changing the functional groups that are attached to its pore walls during the process of synthesis. We have recently reported that an amino-functionalized SBA-15 mesoporous silica exerts a profound influence over the antioxidant release of tocopherol contained in low-density polyethylene polymer films.[6] When tocopherol was preincorporated into such a mesostructure, active polymer films containing the functionalized carrier showed a slower antioxidant release when compared with samples containing either free tocopherol or tocopherol loaded into a purely siliceous SBA-15 substrate.
In general, active materials already on the market are not very widespread in Europe. This is most likely due to some degree of diffidence of the average consumer toward these kinds of new packaging materials. Notwithstanding, food companies, food packaging suppliers and consumers should bear in mind that these new concepts can be a valuable solution to new market requirements such as the emerging social tendency to buy food products at longer time intervals. For this reason, it is highly desirable to continue developing new packaging systems able to maintain the characteristics of fresh food as long as possible.
Future Advances of Active Packaging
The field of food packaging can be considered one of the emerging applications of stimuli-responsive polymer materials. They are an interesting, innovative and challenging class of materials that can adapt to surrounding environments and regulate the transport of molecules as a reaction to external stimuli. To sustain life and maintain biological function, nature requires selectively tailored molecular assemblies and interfaces that provide a specific chemical function and structure as well as a change in their environment. Synthetic polymer systems with very similar attributes are often prepared for a broad range of applications such as controlled-release systems. Recently, stimuli-responsive macromolecular nanostructures have been developed; they are capable of conformational and chemical changes upon reception of external signals such as change in temperature, pH or chemical composition.[7] These materials will permit triggering the release of active compounds only when strictly needed by the system.
Edible Coatings
Edible films and coatings are other strategies widely investigated to meet consumer demands for more natural foods and environmental protection. They can be developed using agricultural commodities and/or wastes of industrial food production, creating additional values from biomass. Edible coatings and films are not studied with the aim of substituting traditional packaging materials. Due to their distinctive properties, they can be used to provide additional functionalities to the food. Food preservation can be therefore improved, and one may also reduce the cost and amount of traditional packaging used.
The biopolymer used to develop edible films and coatings is usually based on hydrocolloids, such as polysaccharides like cellulose, starch, alginates, chitosan, gums, pectins and proteins, from vege-table or animal origin. Their functional properties can be used to modify the barrier to gases and moisture and, in more advanced developments, serve as carriers of food additives and nutrients. Blends or composites with other additives and fillers are also developed to optimize the barrier properties or to control the release kinetics of substance.[8]
The most important property these natural biopolymers must possess is the possibility of forming films with suitable mechanical and barrier properties. The chemical, molecular and supramolecular structures are, therefore, important features of natural biopolymers that permit design of the physicochemical properties of the films as well as the processing technology for the manufacture of the products.
Cellulose and cellulose derivatives, obtained by chemical substitution of some hydroxyl groups along the chain, permit development of films with ionic properties (carboxymethylcellulose) and nonionic cellulose ethers (methylcellulose, hydroxypropylcellulose and hydroxypropyl methylcellulose). Films made by these derivatives are usually very sensitive to water but resistant to oil and fat. They can be used to incorporate functional additives and antimicrobials, such as, for example, nisin or rosemary, and tea extract to reduce lipid oxidative rancidity. Antimicrobial films based on starch and starch derivatives can be obtained by incorporating potassium sorbate or chitosan into the films. However, the presence of these substances could modify the crystalline structures of the films, thus reducing the barrier to gases.
Alginates, biomacromolecules extracted from brown seaweeds, are other interesting polysaccharides due to their capability to react irreversibly with polyvalent metal cations, in particular calcium ions, to produce water-insoluble polymers. For these classes of polysaccharide materials, the release of potassium sorbate or other antimicrobial additives is controlled by modulating the physical properties of the biomacromolecular structure, in particular, through control of the reticular density.
Chitosan, extracted from the shells of crustaceans, is a high-molecular weight cationic polysaccharide that is widely used to make films with antibacterial and anti-fungal activity. It has been extensively used to protect, improve quality and extend the shelf life of fresh and processed foods.
Proteins represent another class of biomacromolecules employed for edible films and coatings. They can have impressive barrier properties to oxygen, carbon dioxide, oil and fats. However, mechanical and water vapor barrier properties of films produced from these materials are inferior to those of synthetic origin.[9]
Both agro- and animal-based proteins such as wheat gluten, corn zein, soy protein, whey proteins, casein, egg white, keratin, collagen, gelatin and myofibrillar proteins have been used to prepare films and edible coatings by using the solvent casting process. Very few publications have reported on the thermoplasticization and the extrusion of these proteins to produce films. The development of extrusion-based technologies with good reproducibility and control over the molecular architecture and spatial conformation of the natural macromolecule is among the main scientific and technological challenges to exploiting the use of protein-based films and coatings.
While thermoplastic starches (TPS) have been widely studied and successfully applied in industry, including blending TPS with other synthetic polymers, the thermoplasticization of proteins has been reported only recently, and it has been investigated on gluten, zein, soy, whey and gelatine.[9]
Several factors must be taken into account when choosing between different possible plasticizers for the development of thermoplastic proteins. The most commonly used plasticizer is glycerol, which is miscible in most cases. Other systems that have been investigated include polyfunctional alcohols, such as sorbitol and propylene glycol, as well as di- and triethanolamine. Heat and shear stresses contribute to the unfolding of the protein in the presence of the plasticizer during the extrusion process. Proper processing conditions such as temperature profile, residence time and screw design are therefore necessary to supply the needed mechanical and thermal energy to the proteins/plasticizer systems. By optimizing protein/plasticizer systems and processing conditions, one could obtain materials with rheological properties suitable for film-blowing technologies.[10]
However, one should be aware that the use of extrusion-based processing could affect the functionalities of antimicrobial compounds embedded in the polymeric matrix. These substances are generally heat sensitive and thermally unstable; thus, they may become inactive during processing, mainly because of the high temperature, high shear rates and high pressure an extruder can reach.[9]
Conclusions
The ongoing scientific and technological interest in developing more efficient ways to use renewable resources will foster the development of novel and advanced substrates based on vegetable biomass. Bio-based materials for added-value applications, such as biomedical uses, drug delivery and advanced packaging systems, could be developed by mimicking the synergy between different biomacromolecules that are naturally assembled in hierarchical structures. Challenging researcher activities are expected to take place in the field of nanocellulose and “nanopapers,” and on protein/polysaccharide self-assembling nanosystems to develop hybrid and supramolecular structures with unique properties not achievable by simple blending or through a “composite” approach. Fully bio-based, edible structures with superhydrophobic, stimuli-responsive properties coupled with high barrier and mechanical properties are just some examples of the possible functional and structural features achievable with a novel material/manufacturing platform based on such biomacromolecules and by using novel nano- and self-assembling technology approaches. However, we must take into account that in nature, biology accomplished much sophisticated construction from molecular bottom up to visible organisms and structures. Construction of hieararchical structures only from a bottom-up approach could be very difficult to exploit at industrial scale. Fusion with the well-developed, top down-type nanofabrication should be taken into consideration for more rapid development of these innovative technologies in added-value applications, included advanced packaging.[11]
Giovanna Buonocore, Ph.D., is currently a researcher at the Institute for Composites and Biomedical Materials (IMCB) – National Research Council.
Salvatore Iannace, Ph.D., is a chemical engineer and senior scientist at the IMCB and serves as adjunct professor at the University of Naples in Italy.
References
1. Lee, D.S., K.L. Yam and L. Piergiovanni. 2008. Food packaging science and technology. CRC Press.
2. Restuccia, D., U.G. Spizzirri, O.I. Parisi, G. Cirillo, M. Curcio, F. Iemma, F. Puoci, G. Vinci and N. Picci. 2010. New EU regulation aspects and global market of active and intelligent packaging for food industry applications. Food Cont 21:1425–1435.
3. Incoronato, A.L., G.G. Buonocore, A. Conte, M. Lavorgna and M.A. Del Nobile. 2010. Active systems based on silver-montmorillonite nanoparticles embedded into bio-based polymer matrices for food packaging applications. J Food Prot 73:2256–2262.
4. Ukmar, T. and O. Planinšek. 2010. Acta Pharm 60:373–385.
5. Mehdi, A., C. Reye and R. Corriu. 2011. Chem Soc Rev 40:563–574.
6. Gargiulo, N., I. Attianese, G.G. Buonocore, D. Caputo, M. Lavorgna, G. Mensitieri and M. Lavorgna. 2012. a-Tocopherol release from active polymer films loaded with functionalized SBA-15 mesoporous silica. Micropor Mesopor Mat, DOI:10.1016/j.micromeso.2012.07.037.
7. Stuart, M.A.C., W.T.S. Hyck, J. Genzer, M. Muller, C. Ober, M. Stamm, G.B. Sukhorukov, I. Szleifer, V.V. Tsukruk, M. Urban, F. Winnik, S. Zauscher, I. Luzinov and S. Minko. 2010. Emerging applications of stimuli-responsive polymer materials. Nature Mater 9:101.
8. Campos, C.A., L.N. Gerschenson and S.K. Flores. 2011. Development of edible films and coatings with antimicrobial activity. Food Bioprocess Technol 4:849–875.
9. Mensitieri, G., E. Di Maio, G. Buonocore, I. Nedi, M. Oliviero, L. Sansone and S. Iannace. 2011. Processing and shelf life issues of selected food packaging materials and structures from renewable resources. Trends Food Sci Technol 22:72–80.
10. Oliviero, M., E. Di Maio and S. Iannace. 2010. Effect of molecular structure on film blowing ability of thermoplastic zein. J Appl Polym Sci 115(1):277–287.
11. Ariga, K., J.P. Hill, M.V. Lee, A. Vinu, R. Charvet and S. Acharya 2008. Challenges and breakthroughs in recent research on self-assembly. Sci Technol Adv Mater 9:014109.
Molecular and Supramolecular Design for Active and Edible Packaging Systems
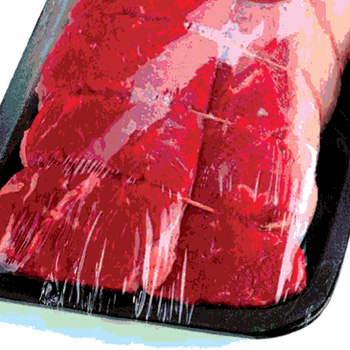