Low-energy electron beam (LEEB) technology was validated in 2020, demonstrating a high level of reduction of Salmonella spp. from spices at industrially relevant throughputs. A letter of no objection from the U.S. Food and Drug Administration (FDA) was recently granted to the breakthrough application. What is it and how does it solve the rising issues of microbial contamination in dry foods?
Microbiological Quality of Dry Foods
Globalization and new consumer trends, demanding fresh, high-quality, minimally processed, ready-to-eat (RTE) foods, have resulted in high industry standards and strict regulations for the food safety of low-moisture foods.1 Fatal foodborne-illness outbreaks, costly lawsuits, and recalls are an issue not only for perishable, high-water-activity RTE products but also for their ingredients, such as spices, herbs, seeds, and grains. The microbial levels on dry food matrices are often extremely high due to potentially low hygiene levels during their growth, harvest, storage, and processing, especially if they include steps such as fermentation or sun drying. Salmonella, Bacillus spp. (including Bacillus cereus), Clostridium perfringens, Shigella, Staphylococcus aureus, as well as insect parts and excrement are among the main pathogens and filth adulterants found in spices.2
Technologies that traditionally tackle such contamination in dry foods are based on treatments using steam (thermal treatment), chemicals (e.g., ethylene oxide), and irradiation. These technologies can sufficiently reduce vegetative pathogens but may be associated with drawbacks such as deterioration of food quality, low efficiency of spore inactivation, high costs, and potential risks to the environment. Thermal treatment introduces water to the dry food facility and to the product, which may cause issues such as increased risk of growth of Listeria or mold after the treatment has been applied. Chemical treatment may leave harmful residues on the end product, alter food quality, and pose a hazard for the operators’ health and safety. Gamma irradiation treatment is outsourced due to the strict safety measures and large footprint of the equipment, which results in lack of control over the product, treatment capacities, and costs. To control bacterial spores, the food industry has to apply more intensive decontamination processing steps (e.g., thermal sterilization) alone or in combination with water activity and pH reduction. Many novel microbial reduction technologies, such as high-pressure processing or UV-C light, are suitable for use on high-moisture foods but may not be effective enough in reducing pathogens on low-moisture foods.3,4
LEEB and Other Irradiation Technologies
Irradiation treatment has been used in food processing for decades to eliminate harmful microorganisms, control infestation by insects, or delay ripening and prevent sprouting of produce. It has become an important way to ensure that foods marketed internationally meet quality and safety standards, especially since some countries limit or prohibit the use of chemicals. The conventional sources of irradiation are X-rays at or below 5 MeV, gamma rays emitted from radioactive isotopes of cobalt-60 or cesium-137, and electron beams at or below 10 MeV.5,6 The gamma-emitting isotopes will decay, leaving radioactive waste that is costly and difficult to dispose of.6 Electron beams and X-rays have no such disadvantage. Due to the high dose rate of electron beam irradiation, only milliseconds are needed to deliver the same effect that takes gamma radiation minutes to hours.7,8 As the electrons are produced by a powered accelerator, the beam can be switched on and off instantly and can also be adjusted for the delivery of the desired dose. This enables a better control and flexibility of the application of this technology.8
Electrons reduce biological contamination by damaging target molecules such as DNA and RNA in two ways: directly, by their energy transfer and breakage of the single and double strands, and indirectly, by generating free radicals by the reaction of the electrons with water molecules or surrounding gases.5,9 This mechanism of inactivation, in comparison to heat treatment or chemicals, allows, at standard processing conditions, for a much better reduction of highly resistant bacterial spores. These spores are the perfect vehicles for spoiling food or infecting humans.10
High-energy electron beam (HEEB) irradiation facilities use electrons with energy between 5 and 10 MeV. This high-beam energy allows the electrons to penetrate several centimeters through the food matrix structure and treat it, even inside its protective packaging.¹¹ This property makes it a great alternative to thermal preservation of minced meat or oysters, where bacteria generally reside everywhere in the material, not only within the first micrometers.
Electrons with energies of 0.3 MeV and lower are defined as low-energy electrons or, by Hayashi, as “soft electrons.”12 Until recently, LEEB was used in the food industry only for the sterilization of food packaging materials. It successfully replaced sterilization with chemicals, which also led to reduced water usage, lower energy consumption, and shorter treatment time while maintaining the same footprint of the equipment.13
The source of low-energy electrons is an electron beam lamp with a tungsten wire in an ultrahigh vacuum. Electrons are accelerated from the wire and emitted through a thin titanium window out of the lamp. Primary low-energy electrons in the air scatter, striking air particles like ozone and causing the emission of secondary electrons, which form an electron cloud. These electrons’ ability to penetrate the food matrix depends on the energy at which they are accelerated. The lower the energy of the electrons, the less penetrability they have—a relationship that impacts not only food quality and the depth of decontamination but also the thickness of the protective shielding needed around the machine. In comparison to HEEB, significantly less shielding is required around a LEEB system to protect workers from the electrons and their by-products.
In 1998, Hayashi stated that “LEEB treatment can decontaminate low-moisture foods without detrimental effects on quality.”12 As most of the microorganisms contaminating dry foods such as spices reside on the surface of the food, the inner matrix does not have to be treated, and the foods’ natural characteristics can be protected or even enhanced.12,14 An absorbed dose is the amount of energy that is deposited in a material by ionizing radiation per unit mass. Irradiation dose is expressed in grays (Gy). In general, low doses (0.05–0.15 kGy) are used to inhibit sprouting and delay the ripening of fresh fruits and vegetables. Medium doses (1.0–10 kGy) are used to control harmful bacteria such as Salmonella spp., Escherichia coli O157:H7, Listeria monocytogenes, and Campylobacter jejuni. Higher doses (10–25 kGy) are generally effective in decontaminating food ingredients, such as spices and herbs. Doses from 25 kGy to 50 kGy sterilize foods for space and hospital diets.15 Due to the limited penetrability of LEEB, high doses can be achieved close to the surface of the grain (e.g., 10–15 kGy). Doses gradually decrease toward the center of the seed. Lower doses, sufficient for insect control, reach the inner parts of the grain (e.g., 0.4–0.5 kGy).16 This offers an unmatched preservation of the quality attributes inside the treated seeds.
Industrial Application of LEEB
According to the Food Safety Modernization Act, every process when introduced into the production as a process preventive control step must be validated and incorporated into a food safety plan at the facility. This means that compelling evidence must be provided that the process constantly delivers the treatment target, for example, the delivery of an irradiation dose that causes a 5-log₁₀ reduction of Salmonella spp. or equivalent in black peppercorns at industrial throughput. LEEB technology was, until recently, studied mainly in regard to the reduction of natural contamination, which does not provide sufficient evidence for validation.¹⁷ In addition, the approaches of measuring the absorbed dose were not provided. Without evidence to link the achieved inactivation of the target pathogen to precise dosimetric values, there was no way to validate the process. Recent developments in both the research of the behavior of vegetative pathogens and thermoresistant bacterial spores in LEEB18-20 and the establishment of approaches toward the dosimetry measurements enabled this technology to be utilized as a process preventive control for whole spices.
When introducing a new pathogen-reduction technology to the market, the confirmation and validation of food safety is paramount, followed by considerations about microbial stability, process capability, and product chemistry. Regulatory agencies must be provided with evidence that the product is meeting safety requirements with a high level of confidence. In the recently validated LEEB processing unit, loose granular product is exposed to a beam of low-energy electrons without protective packaging. To achieve industrially relevant throughputs, it is treated in free fall, between two electron beam lamps (Figure 1). The spice seeds fall spatially separated from one another to provide for a homogenous and sufficient treatment during the short exposure. The irradiation dose that the food surface receives within several milliseconds is around 10–15 kGy. This is sufficient to cause a 5-log₁₀ reduction of Salmonella or equivalent. After the treatment, the material can be fed directly to the final packaging to prevent cross-contamination. Critical processing parameters, such as beam energy and beam current, are recorded, assessed against their limits, and logged at frequent intervals on the device. This information is immediately accessible for the quality manager and regulatory bodies. The in-house solution with continuous process monitoring minimizes potential for food waste, food safety risk, and recalls.21,22
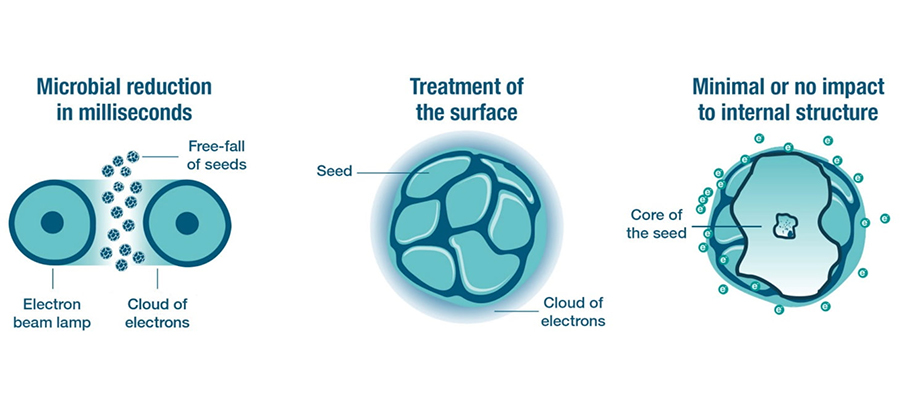
FIGURE 1. LEEB industrial application. The seeds free-fall between low-energy electron beam lamps where each seed is exposed to a cloud of electrons. Due to the low energies of the electrons, only the surface of the seed is treated, preserving the internal quality of the seed.
Measurement of Dose
Irradiation dose is one of the critical control parameters in LEEB. A dose that is too small means that the foodstuff is undertreated, while a dose that is too large would exceed the regulatory limits for food irradiation.23 The absorbed dose is measured by radiochromic B3 film, following the guidelines and practices for irradiation dosimetry applications according to ISO/ASTM 5181824 and ISO/ASTM 51275:2013.25 B3 film is a preferred dosimetry system for LEEB due to its thinness, which can record the penetrability of the beams. A dosimeter the size of a grain was developed. A special scanner can quantify and relate the absorbance in the optical spectrum of the film to the absorbed dose in the simulated grain.26 Grain speed measurements together with Monte Carlo simulations and statistical analysis are used to quantify the absorbed doses.
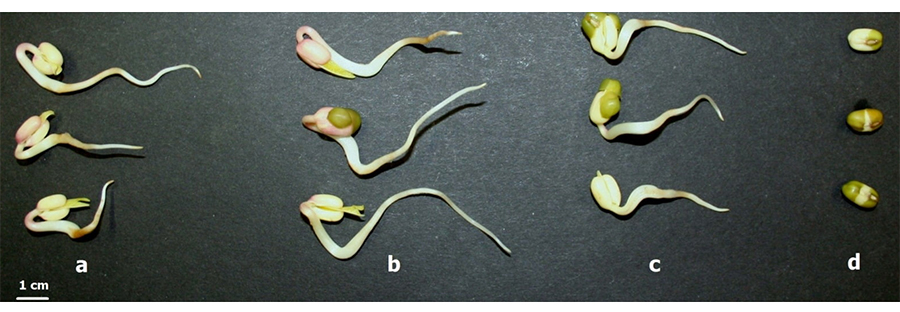
FIGURE 2. Effects of LEEB on mung bean seed germination versus high-energy electrons: (a) untreated; (b) low-energy electron beam treated at 140 keV; (c) LEEB-treated at 200 keV; (d) HEEB-treated at 10 MeV.
Effects of LEEB on Microbial Control
The efficacy of the LEEB technology was tested on black peppercorns with three types of contamination: natural bio-burden, nonpathogenic surrogate for spores of B. cereus (thermoresistant spores of Geobacillus stearothermophilus ATCC 7953), and nonpathogenic surrogate for Salmonella (Enterococcus faecium NRRL B-2354). The tests revealed that natural contamination can be reduced from 8-log₁₀ to 4-log₁₀ in LEEB-treated samples. Naturally present aerobic spores were reduced more than 3-logs and anaerobes more than 4-logs. Yeasts and molds were reduced from 3-log₁₀ initially to less than 1-log₁₀. LEEB-treated pepper was microbiologically stable due to the absence of water and chemicals during the process. LEEB treatment of black peppercorns inoculated with thermoresistant spores of G. stearothermophilus and B. subtilis resulted in noticeable reductions of 4-log₁₀ and 3.5-log₁₀, respectively. Moreover, the extensive validation study has proven that the technology can deliver the doses that reduce Salmonella by 5-log₁₀ (>99.999%) in black peppercorns. For the validation experiments, E. faecium was selected as a nonpathogenic surrogate organism to avoid contamination risks in industrial plants.21
Effects of LEEB on Food Quality Parameters
Every decontamination process has an impact on the food material it treats. What differs among the technologies is the magnitude of the changes. Exposure to temperature and oxidizing agents is an important consideration in production of fresher, minimally processed products. Thermally sensitive vitamins, enzyme activity, and other bioactive constituents are among the most sensitive indicators of the decontamination process. As a nonthermal and surface treatment, LEEB has a minimal impact on the quality attributes of foods, such as aroma and taste. Kotilainen et al.27 studied the impact of different treatments on the quality properties of spices. The study compared the effects of LEEB and the effects of steam treatment on the moisture, color, essential oil yield, and volatile compounds in black peppercorn and coriander seeds. It was observed that the treatment with LEEB can preserve all studied parameters better than steam treatment.
Profound evidence of quality preservation was obtained with mung beans (Vigna radiata), specifically for the preservation of embryo and enzymes responsible for sprouting (Figure 2). Mung bean seeds were treated with HEEB and LEEB prior to germination. Since the low-energy electrons dissipated before they reached the embryo of the seed, the embryo remained undamaged, and the seed was able to germinate. Seeds treated with HEEB were unable to germinate, as the electrons penetrated through the seed and negatively impacted the embryo.27 In 2003, Kikuchi et al.14 recommended LEEB treatment over gamma irradiation for soybean decontamination because it induced minimal or no quality deterioration, since the electrons do not reach the internal matrix.
Effects of LEEB on Pest Control
LEEB treatment is also a viable alternative to fumigation as a pre- or poststorage disinfestation treatment for control of grain pests. Low doses of irradiation must reach the part of the grain where the immature life stages (eggs, larvae, pupae) reside. Tests where rice grains were artificially infested with two rice pests (Rhyzopertha dominica and Sitophilus oryzae) resulted in a promising outcome. While a significant number of adults developed in the untreated transport and processing of control samples, no development was observed in any of the LEEB-treated samples. This indicates that the applied doses were sufficient to reduce the two target insects developing inside rice grains.16
Other Food Applications and Regulatory Status
Spices, edible seeds, culinary herbs, and dehydrated vegetable seasonings are currently listed as low-moisture foodstuffs, which FDA allows to be treated with irradiation for microbial control. When these are used as ingredients, irradiation labeling is not required. Due to the increased number of foodborne-illness recalls and outbreaks in raw flour products such as cookie dough, there is a growing demand for decontamination treatment of cereals. Cereal grains are well suited for treatment with LEEB technology. However, for the moment, there are no established regulations in place for treatment of cereal grains for microbial control. The same applies to pulses and nuts, including almonds, which are also often associated with foodborne illnesses. Food irradiation regulations do not include nonfood products, such as pet food, feed, and pharmaceuticals. For example, U.S. regulation 21 C.F.R. 579 allows the irradiation of pet food up to 50 kGy.28
Nonfood products such as feed, pet food, vegetable and agricultural seeds, and pharmaceuticals are outside the food irradiation regulatory framework. In most regions, irradiation of nonfood is authorized without regulatory restrictions. Therefore, nonfood products are suitable for treatment with LEEB.23
Conclusion
LEEB technology is a validated microbial control solution that sufficiently eliminates human pathogenic bacteria in dry foodstuffs, including thermoresistant bacterial spores, while preserving the natural richness of the food. LEEB offers benefits such as being chemical-free, water-free, radioactive material-free, in-house, compact, and agile, with 24/7 availability. The letter of no objection from FDA has confirmed the readiness of this technology to provide an attractive alternative to conventional solutions with a state-of-the-art pathogen contamination control approach for the 21st century.
References
- http://www.fao.org/3/a-y3696e.pdf.
- FDA. 2017. “Risk Profile: Pathogens and Filth in Spices.” Center for Food Safety and Applied Nutrition, U.S. Department of Health and Human Services.
- Gezai, A. 2019. “Review on High-Pressure Processing of Foods.” Cogent Food Agric 5: 1.
- Guerrero-Beltrmiddle, J.A. 2004. “Advantages and Limitations on Processing Foods by UV Light.” Food Sci Technol Int 10(3): 137–147.
- Tahergorabi, R. 2012. “Application of Electron Beam to Inactivate Salmonella in Food: Recent Developments.” Food Res Int 45: 685–694.
- Silindir, M., et al. 2009. “Sterilization Methods and the Comparison of E-Beam Sterilization with Gamma Radiation Sterilization.” FABAD J Pharm Sci 34: 43–53.
- Fan, X.T., et al. 2017. “Comparison of Gamma and Electron Beam Irradiation in Reducing Populations of E. coli Artificially Inoculated on Mung Bean, Clover and Fenugreek Seeds, and Affecting Germination and Growth of Seeds.” Radiat Phys Chem 130: 306–315.
- Urgiles, E., et al., “Electron Beam Irradiation for Microbial Reduction on Spacecraft Components,” in Proceedings of the 2007 IEEE Aerospace Conference (Big Sky, MT: IEEE, 2007), 1–15.
- Lung, H.M., et al. 2015. “Microbial Decontamination of Food by Electron Beam Irradiation.” Trends Food Sci Technol 44: 66–78.
- Reineke, K., et al. 2020. “Endospore Inactivation by Emerging Technologies: A Review on Target Structures and Inactivation Mechanisms.” Ann Rev Food Sci Technol 11.
- Jaczynski, J., et al. 2003. “Microbial Inactivation and Electron Penetration in Surimi Seafood during Electron Beam Processing.” J Food Sci 68: 1788–1792.
- Hayashi, T. 1998. “Decontamination of Dry Food Ingredients with ‘Soft Electrons’ (Low-Energy Electrons).” Jarq-Jpn Agr Res Q 32: 293–299.
- https://www.tetrapak.com/solutions/packaging/filling-machines/tetra-pak-e3-ebeam.
- Kikuchi, O.K. 2003. “Efficacy of Soft-Electron (Low-Energy Electron Beam) for Soybean Decontamination in Comparison with Gamma Rays.” J Food Sci 68: 649–652.
- WHO. 1999. “High-Dose Irradiation: Wholesomeness of Food Irradiated with 10 kGy.” World Health Organization, Geneva, Switzerland, Technical Report Series 890: 2–160.
- Follett, P., “Advances in Insect Pest Management in Postharvest Storage of Cereals: Irradiation Treatment with X-ray and Electron Beam Machine Sources for Disinfestation,” in Advances in Postharvest Management of Cereals and Grains (Cambridge: Burleigh Dodds Science Publishing, 2020), 319–338.
- Rahman, M.S., et al. 2006. "Inactivation of Cells and Spores of Bacillus subtilis Using Low Energy Pulsed Electron Beam." Jpn J Appl Phys 2(45): L881–L883.
- Zhang, Y., et al. 2018. “Geobacillus and Bacillus Spore Inactivation by Low Energy Electron Beam Technology: Resistance and Influencing Factors.” Front Microbiol 9: 2720.
- Zhang, Y., et al. 2020. “Role of DNA Repair in Bacillus subtilis Spore Resistance to High Energy and Low Energy Electron Beam Treatments.” Food Microbiol 87.
- Moeller, R., et al. 2008. “Roles of the Major, Small, Acid-Soluble Spore Proteins and Spore-Specific and Universal DNA Repair Mechanisms in Resistance of Bacillus subtilis Spores to Ionizing Radiation from X-Rays and High-Energy Charged-Particle Bombardment.” J Bacteriol 190: 1134–1140.
- https://assetcdn.buhlergroup.com/asset/874601345621/5362f9d4a33c4e99b05d32a623d51241.
- http://iiaglobal.com/news/decontamination-dry-food-ingredients-low-energy-electron-beam-world-first/.
- https://www.accessdata.fda.gov/scripts/cdrh/cfdocs/cfCFR/CFRSearch.cfm?fr=179.26.
- ISO/ASTM51818-20, Standard Practice for Dosimetry in an Electron Beam Facility for Radiation Processing at Energies Between 80 and 300 keV (West Conshohocken, PA: ASTM International, 2019).
- ISO/ASTM51275-13, Standard Practice for Use of a Radiochromic Film Dosimetry System (West Conshohocken, PA: ASTM International, 2012).
- Helt-Hansen, J., et al. 2005. “Dose Response of Thin-Film Dosimeters Irradiated with 80–120 keV Electrons.” Radiat Phys Chem 74: 341–353.
- Kotilainen, H., et al., “Effects of Low-Energy Electron Beam (LEEB) Treatment on Physicochemical Attributes of Black Pepper and Coriander,” in Innovative Food Processing Technologies (Amsterdam: Elsevier, 2021), 79–100.
- https://www.accessdata.fda.gov/scripts/cdrh/cfdocs/cfCFR/CFRSearch.cfm?CFRPart=579&showFR=1.
The author thanks Heidi Kotilainen, food regulatory and quality manager, Matthew Murdoch, Ph.D., LEEB dosimetry expert, and Barbora Dubovcova, food safety lead for the LEEB business, Bühler AG, Uzwil, Switzerland, for their expert contributions to this article.
Larry Keener, CFS, PA, PCQI, is president and CEO of International Product Safety Consultants.
This article was originally published in the April/May 2021 issue of Food Safety Magazine.