Vanilla is the second most expensive spice, next to saffron, and is widely used as a flavoring ingredient in the food, beverage, cosmetic, pharmaceutical and tobacco industries. Vanilla extract is the most common form of vanilla used today, and comes in two forms, natural and artificial.
Pure vanilla extract is made by soaking at least 13.35 ounces of vanilla beans in a gallon solution containing a minimum of 35% ethyl alcohol in water. There are two types of artificial vanilla flavorings, which always contain vanillin that is synthesized from cheap raw material such as guaiacol, eugenol or lignin, a natural polymer found in wood; and/or ethyl vanillin that is added, which is another artificially produced vanilla compound that has three times the flavor strength of vanillin.[1]
The production of vanilla beans is quite expensive, since it is a very labor intensive process and harvesting takes place 2 to 3 years after planting. This drives the price of natural vanilla extract to about three to five times higher than artificial vanilla preparations. Due to quality, price concerns and economically motivated frauds, it is important to differentiate between natural and artificial forms of vanilla extracts. Apart from vanillin, natural vanilla extracts have 4-hydroxybenzaldehyde, which is absent in artificial vanilla flavorings. This compound can be used as a marker ion to rapidly differentiate between natural and artificial vanilla preparations.[2]
A variety of analytical techniques such as gas chromatography (GC)/mass spectrometry (MS), liquid chromatography (LC)/MS, headspace GC/MS, LC/ultraviolet (UV) and stable isotope ratio analysis have been used to differentiate between natural and artificial vanilla flavorings.[3] These measurement techniques are either expensive or time consuming, or both, and require extensive method development and sample preparation. In this work, we demonstrated that the AxION® Direct Sample Analysis™ (DSATM) system integrated with the AxION 2 Time-of-Flight (TOF) mass spectrometer can be used for rapid differentiation between artificial and natural vanillin extracts, with no chromatography or sample preparation, and within a few seconds.
Experimental
Five natural and five artificial or imitation vanilla extracts were purchased from a local supermarket. All vanilla extracts were analyzed using the AxION 2 DSA/TOF system and required no sample preparation. Ten microliters of each sample was pipetted directly onto the stainless mesh of the AxION DSA.
DSA/TOF MS Conditions:
• Corona current of 5 μA
• DSA heater temperature of 300 °C
• Auxiliary gas (N2) pressure of 80 psi,
• Drying gas (N2) flow of 3 L/min and drying gas (N2) temperature of 25 °C
The AxION 2 TOF MS was run in negative ionization mode with flight voltage of 5000 V. The capillary exit voltage was set to -120 V for the analysis. Mass spectra were acquired in a range of m/z 50–700 at an acquisition rate of 5 spectra/s. Total analysis time per sample was 15 s. To obtain higher mass accuracy, the AxION 2 TOF instrument was calibrated by infusing a calibrant solution into the DSA source at 10 μL/min.
Results
All 10 vanilla extracts were analyzed by the AxION DSA/TOF. Figures 1 and 2 show the mass spectra for one of the natural vanilla extracts and one of the artificial vanilla extracts, respectively. The mass spectra shows the presence of vanillin in both extracts but 4-hydroxybenzaldehyde was present only in the natural vanilla extracts. This data shows that 4-hydroxybenzaldehyde can be used as a marker compound to distinguish between natural and artificial vanilla extracts using DSA/TOF. All mass measurements showed good mass accuracy with an error of less than 5 ppm.
Similar data were obtained for the other four natural and two of the artificial vanilla extracts. The ingredient labels for the other two artificial vanilla extracts showed the presence of benzoic acid as a preservative. Benzoic acid has the same empirical formula as 4-hydroxybenzaldehyde and therefore it is observed, in the spectra of these extracts, at the same mass as 4-hydroxybenzaldehyde. In order to distinguish between the presence of benzoic acid and 4-hydroxybenzaldehyde in artificial vanilla extracts, fragment ions were generated using collisionally induced dissociation at the capillary exit.
The mass spectrum of a 10 ppm standard of benzoic acid, under these conditions, showed the presence of an ion at m/z 77.0397 Da which corresponds to [M-H-CO2]- ion. This ion was not present however in the corresponding spectrum for a 10 ppm standard of 4-hydroxybenzaldehyde. A mass spectrum of one of the two artificial vanilla extracts is shown in Figure 3. An ion at m/z 121.0368 Da and an ion at m/z 77.0397 Da are easily identifiable confirming the presence of benzoic acid. Furthermore, an ion at m/z 165.0557 Da indicates the presence of ethyl vanillin confirming that this sample is an artificial vanilla extract.
Conclusion
This work shows the utility of the AxION DSA/TOF for rapid differentiation between natural and artificial vanilla extracts for determination of food fraud. This approach showed the presence or absence of 4-hydroxybenzaldehyde in vanillin extracts can be used to distinguish between natural and artificial vanilla extracts. The presence of benzoic acid in some of the artificial extracts can be distinguished from the presence of 4-hydroxybenzaldehyde by monitoring its characteristic fragment ion [ M-H-CO2]-. The presence of ethyl vanillin in some of the artificial extracts can also be used to distinguish further between artificial and natural vanilla extracts. Mass accuracy of all measurements was less than 5 ppm with external calibration. All sample analysis time, was done with no chromatography or sample preparation, at 15 seconds per sample.
In comparison to other established techniques such as LC/MS and GC/MS, this application will improve laboratory productivity and decrease costs and analysis time.
References
1. Herrmann A. and M. Stockli. 1982. J Chrom 246:313–316.
2. Thompson D. R. and J.T. Hoffman. 1988. J Chrom 438:369–382.
3. Cicchetti, E. and E. Chaintreau E. 2009. J Sep Sci 32:3043–3052.
For more information, please visit www.perkinelmer.com.
Rapid Differentiation between Natural and Artificial Vanilla Flavorings for Determining Food Fraud
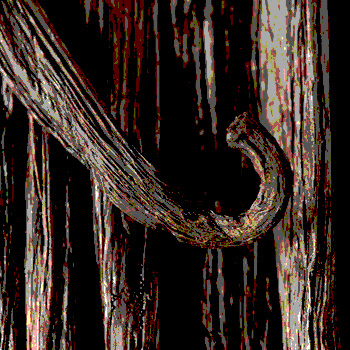